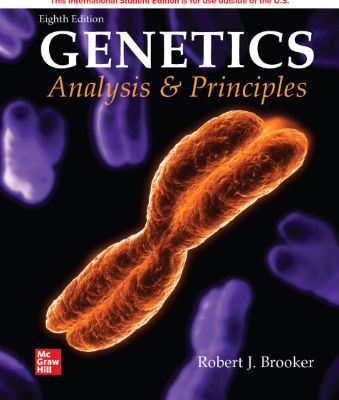
Lýsing:
Genetics: Analysis and Principles is a one-semester, introductory genetics textbook that takes an experimental approach to understanding genetics. By weaving one or two experiments into the narrative of each chapter, students can simultaneously explore the scientific method and understand the genetic principles that have been learned from these experiments. The pedagogy of Genetics: Analysis and Principles has been designed to foster student learning.
Annað
- Höfundar: Robert Brooker, Eric Widmaier, Linda Graham, Peter Stiling
- Útgáfa:8
- Útgáfudagur: 2023-09-12
- Hægt að prenta út 2 bls.
- Hægt að afrita 2 bls.
- Format:ePub
- ISBN 13: 9781266300080
- Print ISBN: 9781266135828
- ISBN 10: 1266300082
Efnisyfirlit
- Table of Contents and Preface
- Cover Page
- Title Page
- Copyright Information
- Brief Contents
- unit I. Introduction
- unit II. Patterns of Inheritance
- unit III. Molecular Structure AND Replication of the Genetic Material
- unit IV. Molecular Properties of Genes
- unit V. Genetic Technologies
- unit VI. Genetic Analysis of Individuals and Populations
- Table of Contents
- unit I. Introduction 1
- unit II. Patterns of Inheritance 21
- unit III. Molecular Structure AND Replication of the Genetic Material 217
- unit IV. Molecular Properties of Genes 298
- unit V. Genetic Technologies 530
- unit VI. Genetic Analysis of Individuals and Populations 631
- About the Author
- Dedication
- Preface
- How We Evaluated Your Needs
- Significant Content Changes in the Eighth Edition
- Examples of Specific Content Changes to Individual Chapters in the 8th Edition
- Suggestions Welcome!
- Acknowledgments
- Reviewers
- Connect
- Instructors The Power of Connections
- Students Get Learning that Fits You
- Connect® Remote Proctoring & Browser-Locking Capabilities
- Chapter 1: Overview of Genetics
- Chapter 1 Introduction
- Overview of Genetics
- 1.1 The Molecular Expression of Genes
- Living Cells Are Composed of Biochemicals
- Each Cell Contains Many Different Proteins That Determine Cell Structure and Function
- DNA Stores the Information for Protein Synthesis
- The Information in DNA Is Accessed During the Process of Gene Expression
- 1.2 The Relationship Between Genes and Traits
- The Molecular Expression of Genes Leads to an Organism’s Traits
- Inherited Differences in Traits Are Due to Genetic Variation
- Traits Are Governed by Genes and by the Environment
- During Reproduction, Genes Are Passed from Parent to Offspring
- The Genetic Composition of a Species Evolves from Generation to Generation
- 1.3 Fields of Genetics
- Model Organisms Are Species That Are Studied by Many Different Researchers
- Transmission Genetics Explores the Inheritance Patterns of Traits as They Are Passed from Parents to Offspring
- Molecular Genetics Focuses on a Biochemical Understanding of the Hereditary Material
- Population Genetics Is Concerned with Genetic Variation and Its Role in Evolution
- 1.4 The Science of Genetics
- Genetics Is an Experimental Science
- Genetic TIPS Will Help You to Improve Your Problem-Solving Skills
- 1.5 Gender Identity Versus Sex
- Gender Identity Is a Person’s Perception of Their Own Gender
- Sex Is Rooted in Anatomical and Cellular Characteristics
- Different Relationships May Occur Between Gender Identity and Sex
- Sexual Orientation Is a Person’s Attraction to Someone Else
- 1.6 The Meaning of Normal in Genetics
- In Genetics, Normal Means Common
- Rare Genetic Variation May Be Beneficial, Neutral, or Detrimental
- Chapter 1 Review
- Key Terms
- Chapter Summary
- Problem Sets & Insights
- Chapter 1 Introduction
- Chapter 2 Introduction
- Chromosome Transmission During Cell Division and Sexual Reproduction
- 2.1 General Features of Chromosomes
- Eukaryotic Chromosomes Are Examined Cytologically to Prepare a Karyotype
- Eukaryotic Chromosomes Are Inherited in Sets
- 2.2 Cell Division
- Bacteria Reproduce Asexually by Binary Fission
- Eukaryotic Cells Advance Through a Cell Cycle to Produce Genetically Identical Daughter Cells
- 2.3 Mitosis and Cytokinesis
- The Mitotic Spindle Apparatus Organizes and Sorts Eukaryotic Chromosomes
- The Transmission of Chromosomes During the Division of Eukaryotic Cells Requires a Process Known as Mitosis
- 2.4 Meiosis
- Meiosis Produces Cells That Are Haploid
- 2.5 Sexual Reproduction
- In Animals, Spermatogenesis Produces Four Haploid Sperm Cells and Oogenesis Produces a Single Haploid Egg Cell
- Plant Species Alternate Between Haploid (Gametophyte) and Diploid (Sporophyte) Generations
- 2.6 Sex Chromosomes and Sex Determination
- Sex Differences May Depend on the Presence of Sex Chromosomes or on the Number of Sets of Chromosomes
- Sex Differences May Depend on the Environment
- Dioecious Plant Species Have Opposite Sexes
- Chapter 2 Review
- Key Terms
- Chapter Summary
- Problem Sets & Insights
- Chapter 3 Introduction
- 3.1 Mendel’s Study of Pea Plants
- Sexual Reproduction in Pea Plants Occurs Via Pollination and Fertilization
- Pea Plants Can Reproduce by Cross-Fertilization or Self-Fertilization
- Mendel Studied Seven Characteristics That Differed Among Strains of Pea Plants
- 3.2 Law of Segregation
- A 3:1 Phenotypic Ratio Is Consistent with the Law of Segregation
- A Punnett Square Can Be Used to Predict the Outcome of Crosses and Self-Fertilization Experiments
- 3.3 Law of Independent Assortment
- Mendel’s Two-Factor Crosses Led to the Law of Independent Assortment
- Independent Assortment Promotes Genetic Diversity
- A Punnett Square Can Be Used to Solve Independent Assortment Problems
- The Forked-Line and Multiplication Methods Can Also Be Used to Solve Independent Assortment Problems
- 3.4 The Chromosome Theory of Inheritance
- The Chromosome Theory of Inheritance Relates the Behavior of Chromosomes to the Mendelian Inheritance of Traits
- The Law of Segregation Is Explained by the Separation of Homologs During Meiosis
- The Law of Independent Assortment Is Explained by the Random Alignment of Homologs During Meiosis
- 3.5 Studying Inheritance Patterns in Humans
- 3.6 Probability and Statistics
- Probability Is the Likelihood That an Outcome Will Occur
- The Product Rule Is Used to Predict the Probability of Independent Outcomes
- The Binomial Expansion Equation Is Used to Predict the Probability of an Unordered Combination of Outcomes
- The Chi Square Test Is Used to Test the Validity of a Genetic Hypothesis
- Chapter 3 Review
- Key Terms
- Chapter Summary
- Problem Sets & Insights
- Chapter 4 Introduction
- 4.1 Overview of Mendelian Inheritance Patterns
- 4.2 Dominant and Recessive Alleles
- Wild-Type Alleles Are Common in a Population
- Recessive Mutant Alleles Often Cause a Reduction in the Amount or Function of the Coded Proteins
- Dominant Mutant Alleles Usually Exert Their Effects in One of Three Ways
- Traits May Skip a Generation Due to Incomplete Penetrance and Vary in Their Expressivity
- 4.3 Environmental Effects on Gene Expression
- 4.4 Incomplete Dominance, Heterozygote Advantage, and Codominance
- Incomplete Dominance Occurs When Two Alleles Produce an Intermediate Phenotype
- Our Conclusions About Dominance May Depend on the Level of Examination
- Heterozygote Advantage Occurs When Heterozygotes Have Greater Reproductive Success
- Alleles of the ABO Blood Group Can Be Dominant, Recessive, or Codominant
- 4.5 Genes on Sex Chromosomes
- The Inheritance Pattern of X-Linked Genes Can Be Revealed by Reciprocal Crosses
- Genes Located on Mammalian Sex Chromosomes Can Be Transmitted in an X-Linked, a Y-Linked, or a Pseudoautosomal Pattern
- 4.6 Sex-Influenced and Sex-Limited Inheritance
- Some Traits Are Limited to One Sex
- 4.7 Lethal Alleles
- 4.8 Understanding Complex Phenotypes Caused by Mutations in Single Genes
- Pleiotropy Occurs When the Expression of a Single Gene Has Two or More Phenotypic Effects
- Certain Coat-Color Patterns in Dogs Are Determined by Events During Embryonic Development
- 4.9 Gene Interactions
- Gene Interaction Can Exhibit Epistasis and Complementation
- Feather Coloration in Parakeets Provides an Example of Gene Modification
- Due to Gene Redundancy, Loss-of-Function Alleles May Have No Effect on Phenotype
- Chapter 4 Review
- Key Terms
- Chapter Summary
- Problem Sets & Insights
- Chapter 5 Introduction
- 5.1 Maternal Effect
- The Genotype of the Female Parent Determines the Phenotype of the Offspring for Maternal Effect Genes
- Oocytes Receive Gene Products That Affect Early Developmental Stages of the Embryo
- 5.2 Epigenetics: Dosage Compensation
- Dosage Compensation Results in Similar Levels of Gene Expression Between the Sexes
- Dosage Compensation Occurs in Female Mammals by the Inactivation of One X Chromosome
- Mammals Maintain One Active X Chromosome in Their Somatic Cells
- X-Chromosome Inactivation in Mammals Depends on the X-Inactivation Center and Occurs in Three Phases
- 5.3 Epigenetics: Genomic Imprinting
- The Expression of an Imprinted Gene Depends on the Sex of the Parent from Which the Gene Was Inherited
- The Imprint Is Established During Gametogenesis
- The Imprinting of Genes Is a Molecular Marking Process That Involves DNA Methylation
- Imprinting Plays a Role in the Inheritance of Certain Human Genetic Diseases
- 5.4 Extranuclear Inheritance
- Mitochondria and Chloroplasts Contain Circular Chromosomes with Many Genes
- Extranuclear Inheritance Produces Non-Mendelian Results in Reciprocal Crosses
- The Pattern of Inheritance of Mitochondria and Chloroplasts Varies Among Different Species
- In Most Animals, Mitochondria Are Inherited Via the Egg
- Many Human Diseases Are Caused by Mitochondrial Mutations
- Extranuclear Genomes of Chloroplasts and Mitochondria Evolved from an Endosymbiotic Relationship
- Chapter 5 Review
- Key Terms
- Chapter Summary
- Problem Sets & Insights
- Chapter 6 Introduction
- 6.1 Overview of Linkage
- Bateson and Punnett Discovered Two Genes That Did Not Assort Independently
- 6.2 Relationship Between Linkage and Crossing Over
- Crossing Over May Produce Recombinant Genotypes
- Morgan Provided Evidence for the Linkage of X-Linked Genes and Proposed That Crossing Over Between X Chromosomes Can Occur
- The Likelihood of Crossing Over Between Two Genes Depends on the Distance Between Them
- A Chi Square Test Can Be Used to Distinguish Between Linkage and Independent Assortment
- Research Studies Provided Evidence That Recombinant Offspring Have Inherited a Chromosome That Is the Product of a Crossover
- 6.3 Genetic Mapping in Plants and Animals
- A Testcross Is Conducted to Produce a Genetic Linkage Map
- Three-Factor Crosses Can Be Used to Determine the Order and Distance Between Linked Genes
- Interference Can Influence the Number of Double Crossovers That Occur in a Short Region
- 6.4 Genetic Mapping in Haploid Eukaryotes
- 6.5 Mitotic Recombination
- Chapter 6 Review
- Key Terms
- Chapter Summary
- Problem Sets & Insights
- Chapter 7 Introduction
- 7.1 Overview of Genetic Transfer in Bacteria
- 7.2 Bacterial Conjugation
- Bacteria Can Transfer Genetic Material During Conjugation
- Conjugation Requires Direct Physical Contact
- An F+ Strain Transfers an F factor to an F− Strain During Conjugation
- Bacteria May Contain Different Types of Plasmids
- 7.3 Conjugation and Mapping via Hfr Strains
- Hfr Strains Have an F Factor Integrated into the Bacterial Chromosome
- Hfr Strains Can Transfer a Portion of the Bacterial Chromosome to Recipient Cells Via Conjugation
- A Genetic Map of the E . coli Chromosome Has Been Obtained from Many Conjugation Studies
- 7.4 Bacterial Transduction
- Bacteriophages Transfer Genetic Material from One Bacterial Cell to Another via Transduction
- 7.5 Bacterial Transformation
- Transformation Involves the Uptake of DNA Molecules into Bacterial Cells
- Some Bacterial Species Have Evolved Ways to Take Up DNA from Their Own Species
- 7.6 Medical Relevance of Horizontal Gene Transfer
- Chapter 7 Review
- Key Terms
- Chapter Summary
- Problem Sets & Insights
- Chapter 8 Introduction
- 8.1 Microscopic Examination of Eukaryotic Chromosomes
- 8.2 Changes in Chromosome Structure: An Overview
- 8.3 Deletions and Duplications
- Deletions Can Happen in Different Ways and Tend to Be Detrimental to an Organism’s Phenotype
- Duplications Tend to Be Less Harmful Than Deletions
- Duplications Provide Additional Material for Gene Evolution, Sometimes Leading to the Formation of Gene Families
- Copy Number Variation Is Relatively Common Among Members of the Same Species
- Microscopy and Molecular Techniques Are Used to Identify Deletions and Duplications
- 8.4 Inversions and Translocations
- Inversions Often Occur Without Phenotypic Consequences
- Inversion Heterozygotes May Produce Abnormal Chromosomes Due to Crossing Over
- Translocations Involve Exchanges Between Different Chromosomes
- Individuals with Reciprocal Translocations May Produce Abnormal Gametes Due to the Segregation of Chromosomes
- 8.5 Changes in Chromosome Number: An Overview
- 8.6 Variation in the Number of Chromosomes Within a Set: Aneuploidy
- Aneuploidy Causes an Imbalance in Gene Expression That Is Often Detrimental to the Phenotype of the Individual
- Aneuploidy in Humans Causes Detrimental Phenotypes
- 8.7 Variation in the Number of Sets of Chromosomes
- Variations in Euploidy Occur Naturally in a Few Animal Species
- Variations in Euploidy Can Occur in Certain Tissues Within an Animal
- Variations in Euploidy Are Common in Plants
- 8.8 Natural and Experimental Mechanisms That Produce Variation in Chromosome Number
- Meiotic Nondisjunction Can Produce Aneuploidy or Polyploidy
- Mitotic Nondisjunction or Chromosome Loss Can Produce a Patch of Tissue with an Altered Chromosome Number
- Changes in Euploidy Can Result in Autopolyploidy, Alloploidy, or Allopolyploidy
- Experimental Treatments Can Promote Polyploidy
- Chapter 8 Review
- Key Terms
- Chapter Summary
- Problem Sets & Insights
- Chapter 9 Introduction
- Molecular Structure of DNA and RNA
- 9.1 Identification of DNA as the Genetic Material
- Griffith’s Experiments Indicated That Genetic Material Can Transform Streptococcus
- Avery, MacLeod, and McCarty Showed That DNA Is the Substance That Transforms Bacteria
- Hershey and Chase Provided Evidence That DNA Is the Genetic Material of T2 Phage
- 9.2 Overview of DNA and RNA Structure
- 9.3 Nucleotide Structure
- 9.4 Structure of a DNA Strand
- 9.5 Discovery of the Double Helix
- A Few Key Events Led to the Discovery of the Double-Helix Structure
- Watson and Crick Deduced the Double-Helix Structure of DNA
- 9.6 Structure of the DNA Double Helix
- The Molecular Structure of the DNA Double Helix Has Several Key Features
- DNA Forms Alternative Types of Double Helices
- 9.7 RNA Structure
- Chapter 9 Review
- Key Terms
- Chapter Summary
- Problem Sets & Insights
- Chapter 10 Introduction
- 10.1 Organization of Functional Sites Along Prokaryotic Chromosomes
- 10.2 Structure of Prokaryotic Chromosomes
- The Formation of Chromosomal Loops Helps Make the Bacterial Chromosome More Compact
- Some Archaeal Chromosomes Contain Histone Proteins that Form Nucleosomes
- DNA Supercoiling Further Compacts a Prokaryotic Chromosome
- Chromosome Function Is Influenced by DNA Supercoiling
- 10.3 Organization of Functional Sites Along Eukaryotic Chromosomes
- 10.4 Sizes of Eukaryotic Genomes and Repetitive Sequences
- The Sizes of Eukaryotic Genomes Vary Substantially
- The Genomes of Eukaryotes Contain Sequences That Are Unique, Moderately Repetitive, or Highly Repetitive
- 10.5 Transposition
- McClintock Found That Chromosomes of Corn Plants Contain Loci That Can Move
- Transposable Elements Move by Different Transposition Pathways
- Each Type of Transposable Element Has a Characteristic Pattern of DNA Sequences
- Transposase Catalyzes the Excision and Insertion of Transposons
- Retrotransposons Use Reverse Transcriptase for Retrotransposition
- Transposable Elements May Have Important Influences on Mutation and Evolution
- 10.6 Structure of Eukaryotic Chromosomes in Nondividing Cells
- Linear DNA Wraps Around Histone Proteins to Form Nucleosomes, the Repeating Structural Unit of Chromatin
- Nucleosomes Associate with Each Other in a Zigzag Manner
- Chromatin Is Further Compacted by the Formation of Loop Domains
- Topologically Associated Domains Are Revealed by Chromosome Conformation Capture Methods
- Some Chromatin Is Highly Compacted During Interphase
- In the Cell Nucleus, Each Chromosome Occupies Its Own Distinct Territory
- Chromosome Organization and Structure in the Cell Nucleus has a Four-Level Hierarchy
- 10.7 Structure of Eukaryotic Chromosomes During Cell Division
- Metaphase Chromosomes Are Highly Compacted
- Condensin I and II Promote the Condensation of Metaphase Chromosomes into Radial Loop Arrays
- Cohesin Promotes the Binding of Sister Chromatids to Each Other
- Chapter 10 Review
- Key Terms
- Chapter Summary
- Problem Sets & Insights
- Chapter 11 Introduction
- 11.1 Structural Overview of DNA Replication
- Existing DNA Strands Act as Templates for the Synthesis of New Strands
- 11.2 Bacterial DNA Replication: The Formation of Two Replication Forks at the Origin of Replication
- Bacterial Chromosomes Contain a Single Origin of Replication
- Replication Is Initiated by the Binding of DnaA Proteins to the Origin of Replication
- 11.3 Bacterial DNA Replication: Synthesis of New DNA Strands
- Several Proteins Are Required for DNA Replication at the Replication Fork
- DNA Polymerase Requires an RNA Primer or an Existing DNA Strand and It Synthesizes DNA Only in the 5′ to 3′ Direction
- The Synthesis of the Leading and Lagging Strands Is Distinctly Different
- Certain Enzymes Involved in DNA Replication Bind to Each Other to Form a Complex
- Replication Is Terminated When the Replication Forks Meet at the Termination Sequences
- The Isolation of Mutants Has Been Instrumental to Our Understanding of DNA Replication
- 11.4 Bacterial DNA Replication: Chemistry and Accuracy
- DNA Polymerase III Is a Processive Enzyme That Uses Deoxyribonucleoside Triphosphates
- The High Fidelity of DNA Replication Is Due to Three Mechanisms
- 11.5 Eukaryotic DNA Replication
- Initiation Occurs at Multiple Origins of Replication on Linear Eukaryotic Chromosomes
- Origins of Replication Differ Among Budding Yeast and Other Eukaryotes
- The Origin Recognition Complex Initiates the Process of DNA Replication
- Eukaryotes Have Many Different DNA Polymerases
- Flap Endonuclease Removes RNA Primers During Eukaryotic DNA Replication
- The Ends of Eukaryotic Chromosomes Are Replicated by Telomerase
- Telomere Length May Play a Role in Aging and Cancer
- Chapter 11 Review
- Key Terms
- Chapter Summary
- Problem Sets & Insights
- Chapter 12 Introduction
- Gene Transcription and RNA Modification
- 12.1 Overview of Transcription
- Gene Expression Requires Base Sequences That Perform Different Functional Roles
- The Three Stages of Transcription Are Initiation, Elongation, and Termination
- 12.2 Transcription in Bacteria
- A Promoter Is a Short Sequence of DNA That Is Necessary to Initiate Transcription
- Bacterial Transcription Is Initiated When RNA Polymerase Holoenzyme Binds at a Promoter
- The RNA Transcript Is Synthesized During the Elongation Stage
- Transcription Is Terminated by Either an RNA-Binding Protein or an Intrinsic Terminator
- 12.3 Transcription in Eukaryotes
- Eukaryotes Have Multiple RNA Polymerases That Are Structurally Similar to the Bacterial Enzyme
- Eukaryotic Protein-Coding Genes Have a Core Promoter and Regulatory Elements
- Transcription of Eukaryotic Protein-Coding Genes Is Initiated When RNA Polymerase II and General Transcription Factors Bind to a Promoter Sequence
- Transcriptional Termination Occurs After the 3′ End of the Transcript Is Cleaved Near the Polyadenylation Signal Sequence
- 12.4 RNA Modification
- rRNAs and tRNAs Are Made as Longer Transcripts That Are Cleaved into Smaller Functional Transcripts
- Different Splicing Mechanisms Remove Introns
- Pre-mRNA Splicing Occurs by the Action of a Spliceosome
- Alternative Splicing Regulates Which Exons Occur in a Mature mRNA, Allowing Different Polypeptides to Be Made from the Same Gene
- The Ends of Eukaryotic Pre-mRNAs Have a 5′ Cap and a 3′ Tail
- The Base Sequence of RNA Can Be Modified by RNA Editing
- 12.5 A Comparison of Transcription and RNA Modification in Bacteria, Archaea, and Eukaryotes
- Chapter 12 Review
- Key Terms
- Chapter Summary
- Problem Sets & Insights
- Chapter 13 Introduction
- 13.1 The Genetic Basis for Protein Synthesis
- Garrod Proposed That Some Genes Code for the Production of Enzymes
- Beadle and Tatum’s Experiments with Neurospora Led Them to Propose the One-Gene/One-Enzyme Hypothesis
- 13.2 The Relationship Between the Genetic Code and Protein Synthesis
- During Translation, the Codons in mRNA Provide the Information to Make a Polypeptide with a Specific Amino Acid Sequence
- Exceptions to the Genetic Code Include the Incorporation of Selenocysteine and Pyrrolysine into Polypeptides
- A Polypeptide Has Directionality from Its Amino-Terminus to Its Carboxyl-Terminus
- The Amino Acid Sequences of Polypeptides Determine the Structure and Function of Proteins
- Proteins Are Primarily Responsible for the Characteristics of Living Cells and an Organism’s Traits
- 13.3 Experimental Determination of the Genetic Code
- The Use of RNA Copolymers and the Triplet-Binding Assay Also Helped to Crack the Genetic Code
- 13.4 Structure and Function of tRNA
- The Function of a tRNA Depends on the Specificity Between the Amino Acid It Carries and Its Anticodon
- Common Structural Features Are Shared by All tRNAs
- Aminoacyl-tRNA Synthetases Charge tRNAs by Attaching the Appropriate Amino Acid
- The Wobble Rules Describe Mismatches That Are Allowed at the Third Position in Codon-Anticodon Pairing
- 13.5 Ribosome Structure and Assembly
- Bacterial and Eukaryotic Ribosomes Are Assembled from rRNA and Proteins
- Components of Ribosomal Subunits Form Functional Sites for Translation
- 13.6 Stages of Translation
- The Initiation Stage Involves the Binding of mRNA and the Initiator tRNA to the Ribosomal Subunits
- Changes in the Expression of eIFs Are Associated with Different Forms of Human Cancers
- Polypeptide Synthesis Occurs During the Elongation Stage
- Termination Occurs When a Stop Codon Is Reached in the mRNA
- Bacterial Translation Can Begin Before Transcription Is Completed
- Antibiotics That Inhibit Bacterial Translation Are Used to Treat Bacterial Diseases
- 13.7 Regulation of Translation
- An Iron Response Element Regulates Translation and mRNA Degradation of Particular mRNAs
- 13.8 A Comparison of Translation in Bacteria, Archaea, and Eukaryotes
- Chapter 13 Review
- Key Terms
- Chapter Summary
- Problem Sets & Insights
- Chapter 14 Introduction
- 14.1 Overview of Transcriptional Regulation
- 14.2 Regulation of the lac Operon
- The Phenomenon of Enzyme Adaptation Is Due to the Synthesis of Cellular Proteins
- The lac Operon Codes Proteins Involved in Lactose Metabolism
- The lac Operon Is Regulated by a Repressor Protein
- The Regulation of the lac Operon Allows a Bacterium to Respond to Environmental Change
- The lac Operon Is Also Regulated by an Activator Protein
- Further Studies Have Revealed That the lac Operon Has Three Operator Sites for Lac Repressor
- 14.3 Regulation of the trp Operon
- The trp Operon Is Regulated by a Repressor Protein
- The trp Operon Is Also Regulated by Attenuation
- Inducible Operons Usually Code Catabolic Enzymes, and Repressible Operons Commonly Code Anabolic Enzymes
- 14.4 Translational and Posttranslational Regulation
- Repressor Proteins and Antisense RNA Can Inhibit Translation
- Posttranslational Regulation Can Occur via Feedback Inhibition or Covalent Modifications
- 14.5 Riboswitches
- A Riboswitch Can Regulate Transcription
- A Riboswitch Can Regulate Translation
- Chapter 14 Review
- Key Terms
- Chapter Summary
- Problem Sets & Insights
- Chapter 15 Introduction
- 15.1 Regulatory Transcription Factors and Enhancers
- Structural Features of Regulatory Transcription Factors Allow Them to Bind to DNA
- Regulatory Transcription Factors Recognize Regulatory Elements Within Enhancers
- The Functions of RTFs Can Be Modulated in Three Ways
- 15.2 Chromatin Remodeling, Histone Variants, and Histone Modifications
- Chromatin-Remodeling Complexes Alter the Positions and Compositions of Nucleosomes
- Histone Variants Play Specialized Roles in Chromatin Structure and Function
- The Histone Code Also Controls Gene Transcription
- 15.3 DNA Methylation
- DNA Methylation Occurs on the Cytosine Base and Usually Inhibits Gene Transcription
- DNA Methylation Is Heritable
- 15.4 Gene Activation and Gene Repression
- Eukaryotic Genes Are Flanked by Nucleosome-Free Regions and Well-Positioned Nucleosomes
- The Formation of a PreInitiation Complex Involves the Binding of Activator Proteins That Promote Changes in Nucleosome Position, Changes in Nucleosome Composition, and Histone Modifications
- Formation of an Open Complex, Promoter Escape, Proximal Promoter Pausing, and Histone Eviction Are Key Events During the Elongation Phase of Transcription
- Steroid Hormones Exert Their Effects by Binding to a Regulatory Transcription Factor
- The CREB Protein Is an Example of an Activator That Is Modulated by Covalent Modification
- Different Mechanisms Can Result in Gene Repression
- Chromatin Immunoprecipitation Sequencing Allows Researchers to Determine the Locations Where Specific Proteins Bind Throughout Entire Genomes
- 15.5 A Comparison of Transcriptional Regulation in Bacteria, Archaea, and Eukaryotes
- Chapter 15 Review
- Key Terms
- Chapter Summary
- Problem Sets & Insights
- Chapter 16 Introduction
- 16.1 Overview of Epigenetics
- Different Types of Molecular Changes Underlie Epigenetic Gene Regulation
- Epigenetic Changes May Be Targeted to Specific Genes by Transcription Factors or Non-coding RNAs
- Epigenetic Changes May Be Maintained by cis- or trans-Epigenetic Mechanisms
- Epigenetic Gene Regulation May Occur as a Programmed Developmental Change or Be Caused by Environmental Agents
- 16.2. Heterochromatin: Function, Structure, Formation, and Maintenance
- Heterochromatin Formation Plays Different Functional Roles in Eukaryotic Cells
- Constitutive Heterochromatin and Facultative Heterochromatin Differ in Their Chromosomal Locations and Molecular Features
- A Series of Molecular Events Results in Gene Silencing and Produces Heterochromatin with a Higher-Order Structure
- The Formation of Heterochromatin Involves Nucleation, Spreading, and Barriers
- The Pattern of Heterochromatin in a Given Chromosome Is Passed from Cell to Cell
- Heterochromatin Structure Is Maintained During Chromosome Replication
- Abnormalities in Heterochromatin Formation Underlie Certain Inherited Human Diseases
- 16.3 Epigenetics and Development
- Genomic Imprinting Occurs During Gamete Formation
- X-Chromosome Inactivation in Mammals Occurs During Embryogenesis
- Pioneer Factors Bind Directly to Nucleosomes and Promote Changes in Cell Fate During Embryonic Development
- Trithorax and Polycomb Group Complexes Are Key Regulators of Epigenetic Changes During Development
- 16.4 Paramutation
- Paramutations Occur When One Allele Alters the Expression of Another Allele
- Paramutations Are Due to Epigenetic Changes That Are Transmitted from Parent to Offspring
- 16.5 Epigenetics and Environmental Agents
- Exposure to Environmental Agents at Early Stages of Development May Cause Epigenetic Changes That Affect Phenotype
- The Different Body Types of Queen and Worker Honeybees Depend on Factors in Royal Jelly That Promote Epigenetic Changes
- Flowering in Certain Species of Plants Is Dependent on Vernalization
- Chapter 16 Review
- Key Terms
- Chapter Summary
- Problem Sets & Insights
- Chapter 17 Introduction
- 17.1 Overview of Non-coding RNAs
- ncRNAs Can Bind to Different Types of Molecules
- ncRNAs Can Perform a Diverse Set of Functions
- The Functions of Some ncRNAs Are Understood
- The Emergence of Living Cells May Have Been Preceded by an RNA World
- 17.2 Non-coding RNAs: Effects on Chromatin Structure and Transcription
- 17.3 Non-coding RNAs: Effects on Translation and mRNA Degradation
- RNA Silencing Was Revealed by Effects on Flower Color in Petunias
- RNA Interference Is Mediated by MicroRNAs or Small Interfering RNAs via an RNA-Induced Silencing Complex
- 17.4 Non-coding RNAs: Effects on RNA Modifications
- 17.5 Non-coding RNAs and Protein Targeting
- 17.6 Non-coding RNAs and Genome Defense
- The CRISPR-Cas System Provides Bacteria with Defense Against Bacteriophages
- PIWI-Interacting RNAs Silence Transposable Elements in Animals
- 17.7 Role of Non-coding RNAs in Human Diseases and Plant Health
- ncRNAs Play a Role in Many Forms of Cancer and Other Human Diseases
- miRNAs Play Key Roles in Plant Health
- Chapter 17 Review
- Key Terms
- Chapter Summary
- Problem Sets & Insights
- Chapter 18 Introduction
- 18.1 Virus Structure and Genetic Composition
- Viruses Differ in Their Host Range, Structure, and Genome Composition
- 18.2 Overview of Viral Reproductive Cycles
- Viruses Follow a Reproductive Cycle That Leads to the Synthesis of New Viruses
- Emerging Viruses, such as HIV and Coronavirus, Have Arisen Recently and Are More Likely Than Previous Strains to Cause Infection
- 18.3 Bacteriophage λ Reproductive Cycles
- Phage λ Can Follow a Lysogenic or Lytic Cycle
- The Choice Between the Lysogenic or Lytic Cycle Depends on the Relative Levels of the cII and cro Proteins
- The λ Repressor and Integrase Control the Lysogenic Cycle
- The Lytic Cycle Depends on the Action of the cro Protein
- OR Acts as a Genetic Switch Between the Lysogenic and Lytic Cycles
- 18.4 HIV Reproductive Cycle
- The HIV-1 Genome Has Nine Genes
- HIV RNA Is Reverse Transcribed into Double-Stranded DNA
- Double-Stranded HIV DNA Is Integrated into the Host Genome
- The Production of New HIV Particles Begins with the Synthesis of HIV RNA and Viral Proteins
- HIV Components Are Assembled and Bud from the Host-Cell Plasma Membrane
- Chapter 18 Review
- Key Terms
- Chapter Summary
- Problem Sets & Insights
- Chapter 19 Introduction
- 19.1 Effects of Mutations on Gene Structure and Function
- Gene Mutations Are Molecular Changes in the DNA Sequence of a Gene
- Gene Mutations Can Alter the Coding Sequence Within a Gene
- Gene Mutations Can Occur Outside of a Coding Sequence and Influence Gene Expression
- Gene Mutations Are Also Given Names That Describe How They Affect the Wild-Type Genotype and Phenotype
- Suppressor Mutations Reverse the Phenotypic Effects of Another Mutation
- Changes in Chromosome Structure Can Affect the Expression of a Gene
- Mutations Can Occur in Germ-Line or Somatic Cells
- 19.2 Random Nature of Mutations
- 19.3 Spontaneous Mutations
- Spontaneous Mutations Can Arise by Depurination, Deamination, or Tautomeric Shifts
- Oxidative Stress May Also Lead to DNA Damage and Mutation
- DNA Sequences Known as Trinucleotide Repeats Are Hot Spots for Mutation
- 19.4 Induced Mutations
- Mutagens Alter DNA Structure in Different Ways
- The Mutation Rate Is the Likelihood of a New Mutation
- Testing Methods Can Determine If an Agent Is a Mutagen
- 19.5 DNA Repair
- Damaged Bases Can Be Directly Repaired
- Base Excision Repair Removes a Damaged Base
- Nucleotide Excision Repair Systems Remove Segments of Damaged DNA
- Mismatch Repair Systems Recognize and Correct a Base-Pair Mismatch
- Double-Strand Breaks Can Be Repaired by Homologous Recombination Repair or by Nonhomologous End Joining
- Damaged DNA May Be Replicated by Translesion DNA Polymerases
- 19.6 Homologous Recombination
- The Holliday Model Describes a Molecular Mechanism for the Recombination Process
- The Double-Strand Break Model Has Refined the Molecular Steps of Homologous Recombination
- Several Proteins Facilitate Homologous Recombination
- Gene Conversion May Result from DNA Mismatch Repair or DNA Gap Repair Synthesis
- Chapter 19 Review
- Key Terms
- Chapter Summary
- Problem Sets & Insights
- Chapter 20 Introduction
- Molecular Technologies
- 20.1 Gene Cloning Using Vectors
- Cloning Experiments May Involve Two Kinds of DNA Molecules: Chromosomal DNA and Vector DNA
- Restriction Enzymes Cut DNA into Pieces and DNA Ligase Joins the Pieces Together
- Gene Cloning Involves the Insertion of DNA Fragments into Vectors, Which Are Propagated in Host Cells
- cDNA Can Be Made from mRNA via Reverse Transcriptase
- A DNA Library May Be Constructed Using Genomic DNA or cDNA
- Gibson Assembly Is a Cloning Method for Joining Three or More DNA Fragments
- 20.2 Polymerase Chain Reaction
- Each Cycle of PCR Involves Three Steps: Denaturation, Primer Annealing, and Primer Extension
- Reverse Transcriptase PCR Is Used to Amplify RNA
- Quantitative PCR Is Used to Quantitate the Amount of a Specific Gene or mRNA in a Sample
- 20.3 DNA Sequencing
- 20.4 Gene Editing via CRISPR-Cas Technology
- Genes in Living Cells Can Be Edited Using CRISPR-Cas Technology
- Gene Expression Can Be Altered Using Dead Cas9
- Gene Editing Has Great Potential But Raises Ethical Issues
- 20.5 Blotting Methods to Detect Gene Products
- Northern Blotting Is Used to Detect a Specific RNA
- Western Blotting Is Used to Detect a Specific Protein
- 20.6 Methods for Analyzing DNA- and RNA-Binding Proteins
- The Electrophoretic Mobility Shift Assay Is Used to Determine If a Protein Binds to a Specific DNA Fragment or RNA Molecule
- DNase I Footprinting Shows Detailed Interactions Between a Protein and DNA
- Chapter 20 Review
- Key Terms
- Chapter Summary
- PROBLEM SETS & INSIGHTS
- Chapter 21 Introduction
- 21.1 Uses of Microorganisms in Biotechnology
- Many Important Medicines Are Produced by Recombinant Microorganisms
- Bacteria Are Used as Biological Control Agents
- Microorganisms Can Reduce Environmental Pollutants
- 21.2 Vaccines
- The First Human Vaccine Was Against Smallpox
- Vaccines Are Diverse in the Types of Components They Contain
- Several Different Vaccines Have Been Developed Against COVID-19
- 21.3 Genetically Modified Animals
- The Genomes of Animals Can Be Altered by Gene Modification or Gene Addition
- Gene Knockouts and Knockins Are Produced in Mice to Understand Gene Function and Human Disease
- Using Biotechnology in the Production of Transgenic Livestock Holds Promise
- 21.4 Reproductive Cloning and Stem Cells
- Researchers Have Succeeded in Cloning Mammals from Somatic Cells
- Stem Cells Have the Ability to Divide and Differentiate into Different Cell Types
- Stem Cells Have the Potential to Treat a Variety of Diseases
- 21.5 Genetically Modified Plants
- Transgenic Plants May Have Characteristics That Are Agriculturally Useful
- Transformation of Agrobacterium tumefaciens and Other Methods Are Used to Make Transgenic Plants
- Chapter 21 Review
- Key Terms
- Chapter Summary
- Problem Sets & Insights
- Chapter 22 Introduction
- 22.1 Overview of Chromosome Mapping
- 22.2 Cytogenetic Mapping via Microscopy
- A Goal of Cytogenetic Mapping Is to Determine the Location of a Gene on an Intact Chromosome
- In Situ Hybridization Localizes Genes Along Particular Chromosomes
- 22.3 Linkage Mapping via Crosses
- Molecular Markers Provide Sites for Mapping Experiments
- Linkage Mapping Uses Molecular Markers Such as Microsatellites
- 22.4 Chromosome Walking and Primer Walking
- 22.5 Overview of Genome Sequencing
- Genome Sequencing Involves the Sequencing of Many Fragments of Chromosomal DNA
- Genome Sequences Can Be Produced Using Short-Read and Long-Read DNA Sequencing Methods
- Innovations in DNA Sequencing Have Made It Less Expensive and Faster
- Illumina Sequencing Technology Provides a Short-Read Method for DNA Sequencing
- 22.6 Genome-Sequencing Projects
- The Human Genome Project Was the Largest Genome-Sequencing Project in History
- Many Genome Sequences Have Been Determined
- 22.7 Metagenomics
- Chapter 22 Review
- Key Terms
- Chapter Summary
- Problem Sets & Insights
- Chapter 23 Introduction
- 23.1 Functional Genomics
- A DNA Microarray Can Quantify Gene Transcription at the Whole Genome Level
- RNA Sequencing (RNA-Seq) Is a Newer Method for Identifying Expressed Genes
- Gene Knockout Collections Allow Researchers to Study Gene Function at the Genomic Level
- 23.2 Proteomics
- The Proteome Is Much Larger Than the Genome
- Protein Purification Methods Are Often Used in Proteomics
- Mass Spectrometry Is Used to Identify Proteins
- Protein Microarrays Are Used to Study Protein Expression and Function
- 23.3 Bioinformatics I: Overview of Computer Analyses and Gene Prediction
- Sequence Files Are Analyzed by Computer Programs
- Different Computational Strategies Can Identify Functional Genetic Sequences
- Computer-Based Approaches Can Identify Genes Within a Long Genomic Sequence
- 23.4 Bioinformatics II: Databases
- 23.5 Bioinformatics III: Homology
- The Sequences of Homologous Genes Are Similar to Each Other
- A Database Can Be Searched to Identify Homologous Sequences
- Homologous Genetic Sequences Can Be Aligned to Identify Conserved Sites That Are Likely to Be Functionally Important
- Chapter 23 Review
- Key Terms
- Chapter Summary
- Problem Sets & Insights
- Chapter 24 Introduction
- Medical Genetics
- 24.1 Inheritance Patterns of Genetic Diseases
- A Genetic Basis for a Human Disease May Be Suggested by a Variety of Observations
- Simple Mendelian Inheritance Patterns of Human Diseases May Be Determined via Pedigree Analysis
- Many Genetic Disorders Exhibit Locus Heterogeneity
- 24.2 Detection of Disease-Causing Alleles via Haplotypes and via Genome-Wide Association Studies
- A Haplotype Is a Series of Linked Genetic Variations on a Chromosome
- Haplotype Association Studies Are Conducted to Identify Disease-Causing Alleles
- Haplotype Association Studies Identified the Mutant Gene That Causes Huntington Disease
- The International HapMap Project Is a Worldwide Effort to Identify Haplotypes in Human Populations
- Genome-Wide Association Studies Can Identify Disease-Causing Alleles in Human Populations
- 24.3 Genetic Testing and Screening
- Genetic Testing Is Used to Identify Many Inherited Human Diseases
- Genetic Screening Identifies Genetic Abnormalities at the Population Level
- Genetic Testing Can Be Performed Prior to Birth
- 24.4 Prions
- 24.5 Human Gene Therapy
- Different Gene Therapy Approaches Have the Potential to Treat Human Diseases
- 24.6 Personalized Medicine
- Molecular Profiling Is Increasingly Used to Classify Tumors and Improve Treatment Options
- DNA Microarrays Are Used in the Molecular Profiling of Tumors
- A Patient’s Genotype Is Important in Determining the Proper Dosage of Certain Drugs
- Chapter 24 Review
- Key Terms
- Chapter Summary
- Problem Sets & Insights
- Chapter 25 Introduction
- 25.1 Overview of Cancer
- 25.2 Oncogenes
- Oncogenes Have Gain-of-Function Mutations That May Affect Proteins Involved in Cell Division Pathways
- Genetic Changes in Proto-Oncogenes Convert Them to Oncogenes
- Certain Viruses Cause Cancer by Carrying Oncogenes into Cells
- 25.3 Tumor-Suppressor Genes
- Tumor-Suppressor Genes, such as the rb Gene, Play a Role in Preventing Cancer
- The Vertebrate p53 Gene Is a Master Tumor-Suppressor Gene That Senses DNA Damage
- Tumor-Suppressor Genes Usually Code Proteins That Regulate Cell Division or Maintain Genome Integrity
- Tumor-Suppressor Genes Can Be Silenced by Loss-of-Function Mutations or Chromosome Loss
- Most Forms of Cancer Involve Multiple Genetic Changes Leading to Malignancy
- Inherited Forms of Cancers Are Usually Caused by Defects in Tumor-Suppressor Genes
- 25.4 Role of Epigenetics in Cancer
- Abnormalities in Chromatin Modification Are Common in Cancer Cells
- Epigenetic Changes Associated with Cancer May Arise Because Mutations Occur in Genes That Code Chromatin-Modifying Proteins or Because Environmental Agents Alter the Functions of Chromatin-Modifying Proteins
- 25.5 Cancer Therapeutics
- Many Different Treatment Options Are Available to Combat Cancer
- A Wide Variety of Cancer Drugs Are Aimed at Killing Actively Dividing Cells
- Targeted Drug Therapies Are Usually Aimed at Specific Proteins That Are Produced by Cancer Cells
- Immunotherapy Uses the Immune System or Components from the Immune System to Kill Cancer Cells
- Drug Therapy May Be Aimed at Epigenetic Changes
- Drug Therapy May Be Used to Alter Non-coding RNAs
- Chapter 25 Review
- Key Terms
- Chapter Summary
- Problem Sets & Insights
- Chapter 26 Introduction
- 26.1 Overview of Animal Development
- The Generation of a Body Pattern Depends on Four Types of Cellular Events
- Positional Information Controls How Cells Behave During Development
- Cell-to-Cell Contacts May Activate Signaling Pathways That Lead to Developmental Changes
- The Study of Mutants with Disrupted Developmental Patterns Has Identified Genes That Control Development
- Animal Development Occurs in Four Overlapping Phases
- 26.2 Invertebrate Development
- Drosophila Advances Through Several Developmental Stages to Become an Adult
- The Early Stages of Embryonic Development Determine the Pattern of Structures in the Adult Organism
- The Gene Products of Maternal Effect Genes Are Deposited Asymmetrically into the Oocyte and Establish the Anteroposterior and Dorsoventral Axes at a Very Early Stage of Development
- The Morphogen Bicoid Is a Transcription Factor That Controls the Development of Anterior Structures
- Gap, Pair-Rule, and Segment-Polarity Genes Act Sequentially to Divide the Drosophila Embryo into Segments
- Homeotic Genes Control the Phenotypic Characteristics of Each Segment
- The Developmental Fate of Each Cell in the Nematode Caenorhabditis elegans Is Known
- 26.3 Vertebrate Development
- Homeotic Genes Are Found in Vertebrates
- Researchers Use Reverse Genetics to Understand Hox Gene Function
- Genes That Code Transcription Factors Also Play a Key Role in Cell Differentiation
- 26.4 Plant Development
- Plant Growth Occurs from Meristems Formed During Embryonic Development
- Plant Homeotic Genes Control Flower Development
- 26.5 Sex Determination in Animals
- In Drosophila, Sex Determination Involves a Regulatory Cascade That Includes Alternative Splicing
- In Mammals, the SRY Gene on the Y Chromosome Determines Maleness
- Chapter 26 Review
- Key Terms
- Chapter Summary
- Problem Sets & Insights
- Chapter 27 Introduction
- 27.1 Genes in Populations and the Hardy-Weinberg Equation
- A Population Is a Group of Interbreeding Individuals That Share a Gene Pool
- At the Population Level, Some Genes May Be Monomorphic, but Most Are Polymorphic
- Population Genetics Is Concerned with Allele, Genotype, and Phenotype Frequencies
- The Hardy-Weinberg Equation Can Be Used to Calculate Genotype Frequencies Based on Allele Frequencies
- The Hardy-Weinberg Equation Can Be Used to Detect Evolutionary Change
- 27.2 An Introduction to Microevolution
- 27.3 Overview of Natural Selection
- Natural Selection Is a Process That Depends on Differences in Reproductive Success
- Darwinian Fitness Is a Measure of Reproductive Success
- The General Selection Model Relates Fitness to the Hardy-Weinberg Equation
- Genome-Wide Selection Scans Can Provide Insight Regarding the Effects of Natural Selection
- 27.4 Patterns of Natural Selection
- Directional Selection Favors the Extreme Phenotype
- The General Selection Model Can Be Applied to Directional Selection
- New Alleles That Are Beneficial May Become Fixed in a Population
- Balanced Polymorphisms May Arise as a Result of Heterozygote Advantage or Negative Frequency-Dependent Selection
- Disruptive Selection Favors Multiple Phenotypes in Heterogeneous Environments
- Stabilizing Selection Favors Individuals with Intermediate Phenotypes
- 27.5 Genetic Drift
- 27.6 Migration
- 27.7 Nonrandom Mating
- 27.8 Sources of New Genetic Variation
- Mutations Can Be Deleterious, Neutral, or Beneficial
- The Mutation Rate Is the Likelihood of a New Mutation
- New Genes Are Produced in Eukaryotes via Exon Shuffling
- New Genes Are Acquired via Horizontal Gene Transfer
- Genetic Variation Is Produced via Changes in Repetitive Sequences
- DNA Fingerprinting Is Used for Identification and Relationship Testing
- Chapter 27 Review
- Key Terms
- Chapter Summary
- Problem Sets & Insights
- Chapter 28 Introduction
- 28.1 Overview of Complex and Quantitative Traits
- Many Quantitative Traits Exhibit a Continuum of Phenotypic Variation That Follows a Normal Distribution
- 28.2 Statistical Methods for Evaluating Quantitative Traits
- Statistical Methods Are Used to Evaluate a Frequency Distribution Quantitatively
- Some Statistical Methods Compare Two Variables with Each Other
- 28.3 Polygenic Inheritance
- For Quantitative Traits That Are Polygenic, Each Gene May Contribute to the Trait in an Additive Way
- Polygenic Inheritance and Environmental Factors May Produce a Continuum of Phenotypes
- 28.4 Identification of Genes That Control Quantitative Traits
- QTL Mapping Relies on Linkage to Molecular Markers
- 28.5 Heritability
- Both Genetic Variance and Environmental Variance May Contribute to Phenotypic Variance
- Phenotypic Variance May Also Be Influenced by Interactions and Associations Between Genotype and the Environment
- Heritability Is the Relative Amount of Phenotypic Variance That Is Due to Genetic Variance
- 28.6 Selective Breeding
- Selective Breeding of Species Can Alter Quantitative Traits Dramatically
- Selective Breeding Provides a Way of Estimating Heritability
- Heterosis May Be Explained by Dominance or Heterozygote Advantage
- Chapter 28 Review
- Key Terms
- Chapter Summary
- Problem Sets & Insights
- Chapter 29 Introduction
- 29.1 Overview of Evolution
- Darwin Formulated the Theory of Evolution by Comparing Different Disciplines
- Genetic Variation and Natural Selection Underlie Adaptive Evolution
- 29.2 Identification of Species and Mechanisms of Reproductive Isolation
- Each Species Is Identified Using Characteristics and Histories That Distinguish It from Other Species
- A Species Concept Is a Way to Define What a Species Is and/or Distinguish Different Species
- 29.3 Speciation
- Speciation Usually Occurs via a Branching Process Called Cladogenesis
- Allopatric Speciation Occurs When Populations Become Separated from Each Other
- Hybrid Zones Are Areas Where Separated Populations Can Interbreed
- Sympatric Speciation Occurs Within the Same Geographic Area
- 29.4 Phylogenetic Trees
- Phylogenetic Trees Are Based on Homology
- A Phylogenetic Tree Depicts the Evolutionary Relationships Among Different Species
- A Phylogenetic Tree Can Be Constructed Using a Cladistic or Phenetic Approach
- The Maximum Likelihood Approach and Bayesian Methods Are Also Used to Discriminate Among Possible Phylogenetic Trees
- Phylogenetic Trees Refine Our Understanding of Evolutionary Relationships
- Horizontal Gene Transfer Also Contributes to the Evolution of Species
- 29.5 Molecular Evolution
- Homologous Genes Are Derived from a Common Ancestral Gene
- Genetic Variation at the Molecular Level Is Associated with Neutral Changes in Gene Sequences
- Molecular Clocks Can Be Used to Date the Divergence of Species
- Evolution Is Associated with Changes in Chromosome Structure and Number
- Synteny Groups Contain the Same Groups of Linked Genes
- Chapter 29 Review
- Key Terms
- Chapter Summary
- Problem Sets & Insights
- Appendix A Introduction
- A.1 Methods for Growing Cells
- A.2 Microscopy
- A.3 Separation Methods
- Disruption of Cellular Components
- Centrifugation
- Chromatography and Gel Electrophoresis
- A.4 Methods for Measuring Concentrations of Molecules and Detecting Radioisotopes and Antigens
- Spectroscopy
- Detection of Radioisotopes
- Detection of Antigens by Radioimmunoassay
- Appendix B
- Chapter 1
- Chapter 2
- Chapter 3
- Chapter 4
- Chapter 5
- Chapter 6
- Chapter 7
- Chapter 8
- Chapter 9
- Chapter 10
- Chapter 11
- Chapter 12
- Chapter 13
- Chapter 14
- Chapter 15
- Chapter 16
- Chapter 17
- Chapter 18
- Chapter 19
- Chapter 20
- Chapter 21
- Chapter 22
- Chapter 23
- Chapter 24
- Chapter 25
- Chapter 26
- Chapter 27
- Chapter 28
- Chapter 29
- Glossary
- Index
- Figure 1.1 Text Alternative (Chapter 1)
- Figure 1.4 Text Alternative (Chapter 1)
- Figure 1.5 Text Alternative (Chapter 1)
- Figure 1.6 Text Alternative (Chapter 1)
- Figure 1.7 Text Alternative (Chapter 1)
- Figure 1.10 Text Alternative (Chapter 1)
- Figure 1.11 Text Alternative (Chapter 1)
- Chromosome Transmission Graphic Text Alternative (Chapter 2)
- Figure 2.1 Text Alternative (Chapter 2)
- Figure 2.2a, b Text Alternative (Chapter 2)
- Figure 2.3 Text Alternative (Chapter 2)
- Figure 2.4 Text Alternative (Chapter 2)
- Figure 2.5 Text Alternative (Chapter 2)
- Figure 2.6 Text Alternative (Chapter 2)
- Figure 2.7 Text Alternative (Chapter 2)
- Figure 2.8 Text Alternative (Chapter 2)
- Figure 2.9 Text Alternative (Chapter 2)
- Figure 2.10 Text Alternative (Chapter 2)
- Figure 2.11 Text Alternative (Chapter 2)
- Figure 2.12 Text Alternative (Chapter 2)
- Figure 2.13 Text Alternative (Chapter 2)
- Figure 2.14 Text Alternative (Chapter 2)
- Figure 2.15 Text Alternative (Chapter 2)
- Figure 2.17b Text Alternative (Chapter 2)
- Diagram of a Eukaryotic Cell in Mitosis Graphic Text Alternative (Chapter 2)
- Diagram of Different of Chromosomes Meiosis Graphic Text Alternative (Chapter 2)
- Diagram of a Eukaryotic Cell in Meiosis Graphic Text Alternative (Chapter 2)
- Diagram of a Eukaryotic Cell Divide Graphic Text Alternative (Chapter 2)
- Figure 3.2a, c Text Alternative (Chapter 3)
- Figure 3.3 Text Alternative (Chapter 3)
- Figure 3.4 Text Alternative (Chapter 3)
- Figure 3.5 Text Alternative (Chapter 3)
- Figure 3.6 Text Alternative (Chapter 3)
- Figure 3.7 Text Alternative (Chapter 3)
- Figure 3.8 Text Alternative (Chapter 3)
- Figure 3.9 Text Alternative (Chapter 3)
- Figure 3.10 Text Alternative (Chapter 3)
- Figure 3.11 Text Alternative (Chapter 3)
- Figure 3.12 Text Alternative (Chapter 3)
- Figure 3.13 Text Alternative (Chapter 3)
- Figure 3.14 Text Alternative (Chapter 3)
- Figure 3.15 Text Alternative (Chapter 3)
- Male gametes Graphic Text Alternative (Chapter 3)
- Genotypes are Filled into the Punnett Square Graphic Text Alternative (Chapter 3)
- Punnett Square to Predict the Outcome Graphic Text Alternative (Chapter 3)
- Punnett Square Predicts the Genotypes of the F2 Flies Graphic Text Alternative (Chapter 3)
- Punnett Square Predicts the Results of the Testcross Graphic Text Alternative (Chapter 3)
- Human Pedigree Graphic Text Alternative (Chapter 3)
- With regard to genetics Graphic Text Alternative (Chapter 3)
- Punnett Square for Fur Color in Dogs Graphic Text Alternative (Chapter 3)
- Three Factor Cross Graphic Text Alternative (Chapter 3)
- Human Pedigree Graphic Text Alternative (Chapter 3)
- Human Disorder Characterized Graphic Text Alternative (Chapter 3)
- Figure 4.2 Text Alternative (Chapter 4)
- Figure 4.3a Text Alternative (Chapter 4)
- Figure 4.4c Text Alternative (Chapter 4)
- Figure 4.5 Text Alternative (Chapter 4)
- Figure 4.6 Text Alternative (Chapter 4)
- Figure 4.7c Text Alternative (Chapter 4)
- Figure 4.8 Text Alternative (Chapter 4)
- Figure 4.9 Text Alternative (Chapter 4)
- Figure 4.10 Text Alternative (Chapter 4)
- Figure 4.11b Text Alternative (Chapter 4)
- Figure 4.12 Text Alternative (Chapter 4)
- Figure 4.15b Text Alternative (Chapter 4)
- Figure 4.17 Text Alternative (Chapter 4)
- Figure 4.18 Text Alternative (Chapter 4)
- Figure 4.19 Text Alternative (Chapter 4)
- Punnett Square for Flower Color Graphic Text Alternative (Chapter 4)
- Two Punnett Squares are Possible Graphic Text Alternative (Chapter 4)
- A Pedigree Graphic Text Alternative (Chapter 4)
- Glycosyl Transferase Encoded by H Gene Graphic Text Alternative (Chapter 4)
- Two Pedigrees Graphic Text Alternative (Chapter 4)
- A Trait Determined by a Single Gene Graphic Text Alternative (Chapter 4)
- Involves a Trait Determined by a Single Gene Graphic Text Alternative (Chapter 4)
- Figure 5.1 Text Alternative (Chapter 5)
- Figure 5.2 Text Alternative (Chapter 5)
- Figure 5.4 Text Alternative (Chapter 5)
- Figure 5.5 Text Alternative (Chapter 5)
- Figure 5.6 Text Alternative (Chapter 5)
- Figure 5.7 Text Alternative (Chapter 5)
- Figure 5.8 Text Alternative (Chapter 5)
- Figure 5.9 Text Alternative (Chapter 5)
- Figure 5.10 Text Alternative (Chapter 5)
- Figure 5.11 Text Alternative (Chapter 5)
- Figure 5.13 Text Alternative (Chapter 5)
- Figure 5.14 Text Alternative (Chapter 5)
- Figure 5.15 Text Alternative (Chapter 5)
- Figure 5.16 Text Alternative (Chapter 5)
- Figure 5.17 Text Alternative (Chapter 5)
- Interpreting the Data Graphic Text Alternative (Chapter 5)
- Northern Blot Graphic Text Alternative (Chapter 5)
- Figure 6.1 Text Alternative (Chapter 6)
- Figure 6.2 Text Alternative (Chapter 6)
- Figure 6.3 Text Alternative (Chapter 6)
- Figure 6.4 Text Alternative (Chapter 6)
- Figure 6.5 Text Alternative (Chapter 6)
- Figure 6.6 Text Alternative (Chapter 6)
- Figure 6.7 Text Alternative (Chapter 6)
- Figure 6.8 Text Alternative (Chapter 6)
- Figure 6.9 Text Alternative (Chapter 6)
- Figure 6.10 Text Alternative (Chapter 6)
- Figure 6.11 Text Alternative (Chapter 6)
- Figure 6.12 Text Alternative (Chapter 6)
- Figure 6.13 Text Alternative (Chapter 6)
- The Likelihood of Crossing Graphic Text Alternative (Chapter 6)
- Punnett Square Predicts the Outcome Graphic Text Alternative (Chapter 6)
- A Cell Going through Meiosis Graphic Text Alternative (Chapter 6)
- Three-Factor Crosses Graphic Text Alternative (Chapter 6)
- Testcross by Mating F1 Female Heterozygotes Graphic Text Alternative (Chapter 6)
- Double Cross-Over Occurs Graphic Text Alternative (Chapter 6)
- Two Sets of Homologous Chromosomes Graphic Text Alternative (Chapter 6)
- The Testcross Graphic Text Alternative (Chapter 6)
- A Double Cross-Over Involving 4 Chromatids Graphic Text Alternative (Chapter 6)
- A Double Cross-Over Involving 3 Chromatids Graphic Text Alternative (Chapter 6)
- A Double Cross-Over Involving 2 Chromatids Graphic Text Alternative (Chapter 6)
- A Human Pedigree Graphic Text Alternative (Chapter 6)
- Figure 7.1 Text Alternative (Chapter 7)
- Figure 7.2 Text Alternative (Chapter 7)
- Figure 7.3 Text Alternative (Chapter 7)
- Figure 7.4a Text Alternative (Chapter 7)
- Figure 7.5 Text Alternative (Chapter 7)
- Figure 7.6 Text Alternative (Chapter 7)
- Figure 7.7 Text Alternative (Chapter 7)
- Figure 7.8 Text Alternative (Chapter 7)
- Figure 7.9 Text Alternative (Chapter 7)
- Figure 7.10 Text Alternative (Chapter 7)
- Figure 7.11 Text Alternative (Chapter 7)
- Figure 7.12 Text Alternative (Chapter 7)
- E. Coli Genetic Map Graphic Text Alternative (Chapter 7)
- Conjugation Experiment Graph with Leucine Graphic Text Alternative (Chapter 7)
- Conjugation Experiment Graph with Histidine Graphic Text Alternative (Chapter 7)
- Figure 8.1b, c, d Text Alternative (Chapter 8)
- Figure 8.2 Text Alternative (Chapter 8)
- Figure 8.3 Text Alternative (Chapter 8)
- Figure 8.5 Text Alternative (Chapter 8)
- Figure 8.6 Text Alternative (Chapter 8)
- Figure 8.7 Text Alternative (Chapter 8)
- Figure 8.8 Text Alternative (Chapter 8)
- Figure 8.9 Text Alternative (Chapter 8)
- Figure 8.10 Text Alternative (Chapter 8)
- Figure 8.11 Text Alternative (Chapter 8)
- Figure 8.12 Text Alternative (Chapter 8)
- Figure 8.13a, b Text Alternative (Chapter 8)
- Figure 8.14 Text Alternative (Chapter 8)
- Figure 8.15 Text Alternative (Chapter 8)
- Figure 8.16 Text Alternative (Chapter 8)
- Figure 8.17 Text Alternative (Chapter 8)
- Figure 8.19a, c Text Alternative (Chapter 8)
- Figure 8.21 Text Alternative (Chapter 8)
- Figure 8.22a, b Text Alternative (Chapter 8)
- Figure 8.23 Text Alternative (Chapter 8)
- Figure 8.24a, b, c Text Alternative (Chapter 8)
- Figure 8.26 Text Alternative (Chapter 8)
- Comparative Genomic Hybridization Experiment Graphic Text Alternative (Chapter 8)
- Gene Duplication and Deletion Graphic Text Alternative (Chapter 8)
- Inverted Chromosome Graphic Text Alternative (Chapter 8)
- Inversion Heterozygote Graphic Text Alternative (Chapter 8)
- Two Chromosomes Graphic Text Alternative (Chapter 8)
- A Chromosome with an Inversion Graphic Text Alternative (Chapter 8)
- A Chromosome Graphic Text Alternative (Chapter 8)
- Two Sets of Chromosomes Graphic Text Alternative (Chapter 8)
- A Set of Normal and Abnormal Chromosomes Graphic Text Alternative (Chapter 8)
- Two Normal Chromosomes Graphic Text Alternative (Chapter 8)
- Figure 9.1 Text Alternative (Chapter 9)
- Figure 9.2 Text Alternative (Chapter 9)
- Figure 9.3 Text Alternative (Chapter 9)
- Figure 9.4 Text Alternative (Chapter 9)
- Figure 9.5 Text Alternative (Chapter 9)
- Figure 9.6 Text Alternative (Chapter 9)
- Figure 9.7 Text Alternative (Chapter 9)
- Figure 9.8 Text Alternative (Chapter 9)
- Figure 9.10 Text Alternative (Chapter 9)
- Figure 9.11 Text Alternative (Chapter 9)
- Figure 9.13 Text Alternative (Chapter 9)
- Figure 9.14 Text Alternative (Chapter 9)
- Figure 9.15 Text Alternative (Chapter 9)
- Figure 9.16 Text Alternative (Chapter 9)
- Figure 9.17 Text Alternative (Chapter 9)
- Some proteins bind to DNA Graphic Text Alternative (Chapter 9)
- A methylated cytosine base Graphic Text Alternative (Chapter 9)
- Figure 10.1 Text Alternative (Chapter 10)
- Figure 10.4 Text Alternative (Chapter 10)
- Figure 10.5 Text Alternative (Chapter 10)
- Figure 10.6 Text Alternative (Chapter 10)
- Figure 10.7 Text Alternative (Chapter 10)
- Figure 10.8 Text Alternative (Chapter 10)
- Figure 10.9 Text Alternative (Chapter 10)
- Figure 10.10 Text Alternative (Chapter 10)
- Figure 10.11 Text Alternative (Chapter 10)
- Figure 10.12 Text Alternative (Chapter 10)
- Figure 10.13 Text Alternative (Chapter 10)
- Figure 10.14 Text Alternative (Chapter 10)
- Figure 10.15 Text Alternative (Chapter 10)
- Figure 10.16 Text Alternative (Chapter 10)
- Figure 10.17 Text Alternative (Chapter 10)
- Figure 10.18 Text Alternative (Chapter 10)
- Figure 10.20 Text Alternative (Chapter 10)
- Figure 10.21 Text Alternative (Chapter 10)
- Figure 10.22 Text Alternative (Chapter 10)
- Figure 10.23 Text Alternative (Chapter 10)
- Figure 10.24 Text Alternative (Chapter 10)
- Figure 10.25 Text Alternative (Chapter 10)
- Figure 10.26 Text Alternative (Chapter 10)
- Figure 10.27 Text Alternative (Chapter 10)
- Figure 10.28 Text Alternative (Chapter 10)
- Figure 10.29 Text Alternative (Chapter 10)
- Figure 10.30 Text Alternative (Chapter 10)
- Figure 11.1 Text Alternative (Chapter 11)
- Figure 11.2 Text Alternative (Chapter 11)
- Figure 11.3 Text Alternative (Chapter 11)
- Figure 11.4 Text Alternative (Chapter 11)
- Figure 11.5 Text Alternative (Chapter 11)
- Figure 11.6 Text Alternative (Chapter 11)
- Figure 11.7 Text Alternative (Chapter 11)
- Figure 11.8a Text Alternative (Chapter 11)
- Figure 11.9 Text Alternative (Chapter 11)
- Figure 11.10 Text Alternative (Chapter 11)
- Figure 11.11 Text Alternative (Chapter 11)
- Figure 11.12 Text Alternative (Chapter 11)
- Figure 11.13 Text Alternative (Chapter 11)
- Figure 11.14 Text Alternative (Chapter 11)
- Figure 11.15 Text Alternative (Chapter 11)
- Figure 11.16 Text Alternative (Chapter 11)
- Figure 11.17 Text Alternative (Chapter 11)
- Figure 11.19 Text Alternative (Chapter 11)
- Figure 11.20 Text Alternative (Chapter 11)
- Figure 11.21 Text Alternative (Chapter 11)
- Figure 11.22 Text Alternative (Chapter 11)
- Figure 11.23 Text Alternative (Chapter 11)
- Figure 11.24 Text Alternative (Chapter 11)
- Figure 11.25 Text Alternative (Chapter 11)
- Meselson and Stahl’s Experiment Graphic Text Alternative (Chapter 11)
- Okazaki fragments Graphic Text Alternative (Chapter 11)
- Figure 12.1 Text Alternative (Chapter 12)
- Figure 12.2 Text Alternative (Chapter 12)
- Figure 12.3 Text Alternative (Chapter 12)
- Figure 12.4 Text Alternative (Chapter 12)
- Figure 12.5 Text Alternative (Chapter 12)
- Figure 12.7 Text Alternative (Chapter 12)
- Figure 12.8 Text Alternative (Chapter 12)
- Figure 12.9 Text Alternative (Chapter 12)
- Figure 12.10 Text Alternative (Chapter 12)
- Figure 12.11 Text Alternative (Chapter 12)
- Figure 12.12 Text Alternative (Chapter 12)
- Figure 12.13 Text Alternative (Chapter 12)
- Figure 12.14 Text Alternative (Chapter 12)
- Figure 12.15 Text Alternative (Chapter 12)
- Figure 12.16 Text Alternative (Chapter 12)
- Figure 12.17 Text Alternative (Chapter 12)
- Figure 12.18 Text Alternative (Chapter 12)
- Figure 12.19 Text Alternative (Chapter 12)
- Figure 12.20 Text Alternative (Chapter 12)
- Figure 12.21 Text Alternative (Chapter 12)
- Figure 12.22 Text Alternative (Chapter 12)
- Figure 12.23 Text Alternative (Chapter 12)
- Figure 12.24 Text Alternative (Chapter 12)
- Figure 12.25 Text Alternative (Chapter 12)
- Table 12.2 Graphic Text Alternative (Chapter 12)
- A Northern Blot Graphic Text Alternative (Chapter 12)
- A DNA Gel with 2 Lanes Graphic Text Alternative (Chapter 12)
- RNA Polymerase II Plus TFIID and TFIIB Graphic Text Alternative (Chapter 12)
- Translation of mRNA Graphic Text Alternative (Chapter 13)
- Figure 13.1 Text Alternative (Chapter 13)
- Figure 13.2 Text Alternative (Chapter 13)
- Figure 13.3 Text Alternative (Chapter 13)
- Figure 13.4 Text Alternative (Chapter 13)
- Figure 13.5 Text Alternative (Chapter 13)
- Figure 13.6 Text Alternative (Chapter 13)
- Figure 13.7 Text Alternative (Chapter 13)
- Figure 13.8 Text Alternative (Chapter 13)
- Figure 13.9 Text Alternative (Chapter 13)
- Figure 13.10 Text Alternative (Chapter 13)
- Figure 13.11 Text Alternative (Chapter 13)
- Figure 13.12 Text Alternative (Chapter 13)
- Figure 13.13 Text Alternative (Chapter 13)
- Figure 13.14 Text Alternative (Chapter 13)
- Figure 13.15 Text Alternative (Chapter 13)
- Figure 13.16 Text Alternative (Chapter 13)
- Figure 13.17 Text Alternative (Chapter 13)
- Figure 13.18 Text Alternative (Chapter 13)
- Figure 13.19 Text Alternative (Chapter 13)
- Figure 13.20 Text Alternative (Chapter 13)
- Table 13.1 Graphic Text Alternative (Chapter 13)
- Table 13.5b, c Graphic Text Alternative (Chapter 13)
- The Binding between a Codon and Anticodon Graphic Text Alternative (Chapter 13)
- A Western Blot Graphic Text Alternative (Chapter 13)
- Western Blot Analysis Graphic Text Alternative (Chapter 13)
- Three Northern Blots Graphic Text Alternative (Chapter 13)
- Figure 14.1 Text Alternative (Chapter 14)
- Figure 14.2 Text Alternative (Chapter 14)
- Figure 14.3 Text Alternative (Chapter 14)
- Figure 14.4 Text Alternative (Chapter 14)
- Figure 14.5 Text Alternative (Chapter 14)
- Figure 14.6 Text Alternative (Chapter 14)
- Figure 14.7 Text Alternative (Chapter 14)
- Figure 14.8 Text Alternative (Chapter 14)
- Figure 14.9 Text Alternative (Chapter 14)
- Figure 14.10 Text Alternative (Chapter 14)
- Figure 14.11 Text Alternative (Chapter 14)
- Figure 14.12 Text Alternative (Chapter 14)
- Figure 14.13 Text Alternative (Chapter 14)
- Figure 14.14 Text Alternative (Chapter 14)
- Figure 14.15 Text Alternative (Chapter 14)
- Figure 14.16 Text Alternative (Chapter 14)
- Figure 14.17 Text Alternative (Chapter 14)
- Figure 14.18 Text Alternative (Chapter 14)
- Complementary to the mRNA Graphic Text Alternative (Chapter 14)
- Figure 15.1 Text Alternative (Chapter 15)
- Figure 15.2 Text Alternative (Chapter 15)
- Figure 15.3 Text Alternative (Chapter 15)
- Figure 15.4 Text Alternative (Chapter 15)
- Figure 15.5 Text Alternative (Chapter 15)
- Figure 15.6 Text Alternative (Chapter 15)
- Figure 15.7 Text Alternative (Chapter 15)
- Figure 15.8 Text Alternative (Chapter 15)
- Figure 15.9 Text Alternative (Chapter 15)
- Figure 15.10 Text Alternative (Chapter 15)
- Figure 15.11 Text Alternative (Chapter 15)
- Figure 15.12 Text Alternative (Chapter 15)
- Figure 15.13 Text Alternative (Chapter 15)
- Figure 15.14 Text Alternative (Chapter 15)
- DNA Contains an Upstream Region Graphic Text Alternative (Chapter 15)
- Coding sequence for gene Graphic Text Alternative (Chapter 15)
- A DNA Gel Graphic Text Alternative (Chapter 15)
- Core Promoter Graphic Text Alternative (Chapter 15)
- Pancreatic Cells or Into Kidney Cells Graphic Text Alternative (Chapter 15)
- Electrophoretic Mobility Shift Assay Graphic Text Alternative (Chapter 15)
- Figure 16.1 Text Alternative (Chapter 16)
- Figure 16.2 Text Alternative (Chapter 16)
- Figure 16.3 Text Alternative (Chapter 16)
- Figure 16.4 Text Alternative (Chapter 16)
- Figure 16.5 Text Alternative (Chapter 16)
- Figure 16.6 Text Alternative (Chapter 16)
- Figure 16.7 Text Alternative (Chapter 16)
- Figure 16.8 Text Alternative (Chapter 16)
- Figure 16.9 Text Alternative (Chapter 16)
- Figure 16.10 Text Alternative (Chapter 16)
- Figure 16.11 Text Alternative (Chapter 16)
- Figure 16.12 Text Alternative (Chapter 16)
- Figure 16.13 Text Alternative (Chapter 16)
- Figure 16.14 Text Alternative (Chapter 16)
- Figure 16.15 Text Alternative (Chapter 16)
- Figure 16.17a, b, c Text Alternative (Chapter 16)
- Spermatogenesis and oogenesis Graphic Text Alternative (Chapter 16)
- Figure 17.1a Text Alternative (Chapter 17)
- Figure 17.2 Text Alternative (Chapter 17)
- Figure 17.3 Text Alternative (Chapter 17)
- Figure 17.4 Text Alternative (Chapter 17)
- Figure 17.5 Text Alternative (Chapter 17)
- Figure 17.6 Text Alternative (Chapter 17)
- Figure 17.7 Text Alternative (Chapter 17)
- Figure 17.8 Text Alternative (Chapter 17)
- Figure 17.9 Text Alternative (Chapter 17)
- Figure 17.10 Text Alternative (Chapter 17)
- Figure 17.11b, c, d Text Alternative (Chapter 17)
- Figure 17.12 Text Alternative (Chapter 17)
- Figure 18.1 Text Alternative (Chapter 18)
- Figure 18.2 Text Alternative (Chapter 18)
- Figure 18.3 Text Alternative (Chapter 18)
- Figure 18.4 Text Alternative (Chapter 18)
- Figure 18.7 Text Alternative (Chapter 18)
- Figure 18.8 Text Alternative (Chapter 18)
- Figure 18.9 Text Alternative (Chapter 18)
- Figure 18.10 Text Alternative (Chapter 18)
- Figure 18.11 Text Alternative (Chapter 18)
- Figure 18.12 Text Alternative (Chapter 18)
- Figure 18.13 Text Alternative (Chapter 18)
- Figure 18.14 Text Alternative (Chapter 18)
- Figure 18.15 Text Alternative (Chapter 18)
- Figure 18.16 Text Alternative (Chapter 18)
- Gene Mutations Graphic Text Alternative (Chapter 19)
- Coding Sequence Graphic Text Alternative (Chapter 19)
- Figure 19.1 Text Alternative (Chapter 19)
- Figure 19.2 Text Alternative (Chapter 19)
- Figure 19.4 Text Alternative (Chapter 19)
- Figure 19.6 Text Alternative (Chapter 19)
- Figure 19.7 Text Alternative (Chapter 19)
- Figure 19.8 Text Alternative (Chapter 19)
- Figure 19.9 Text Alternative (Chapter 19)
- Figure 19.10 Text Alternative (Chapter 19)
- Figure 19.11 Text Alternative (Chapter 19)
- Figure 19.12 Text Alternative (Chapter 19)
- Figure 19.13 Text Alternative (Chapter 19)
- Figure 19.14 Text Alternative (Chapter 19)
- Figure 19.15 Text Alternative (Chapter 19)
- Figure 19.16 Text Alternative (Chapter 19)
- Figure 19.17 Text Alternative (Chapter 19)
- Figure 19.18 Text Alternative (Chapter 19)
- Figure 19.19 Text Alternative (Chapter 19)
- Figure 19.20 Text Alternative (Chapter 19)
- Figure 19.21 Text Alternative (Chapter 19)
- Figure 19.22 Text Alternative (Chapter 19)
- Figure 19.23 Text Alternative (Chapter 19)
- Figure 19.24 Text Alternative (Chapter 19)
- Figure 19.25 Text Alternative (Chapter 19)
- Figure 19.26 Text Alternative (Chapter 19)
- Table 19.3 Graphic Text Alternative (Chapter 19)
- Holliday junction Graphic Text Alternative (Chapter 19)
- Figure 20.1 Text Alternative (Chapter 20)
- Figure 20.2 Text Alternative (Chapter 20)
- Figure 20.3 Text Alternative (Chapter 20)
- Figure 20.4 Text Alternative (Chapter 20)
- Figure 20.5 Text Alternative (Chapter 20)
- Figure 20.6 Text Alternative (Chapter 20)
- Figure 20.7a Text Alternative (Chapter 20)
- Figure 20.8 Text Alternative (Chapter 20)
- Figure 20.9 Text Alternative (Chapter 20)
- Figure 20.10 Text Alternative (Chapter 20)
- Figure 20.11 Text Alternative (Chapter 20)
- Figure 20.12 Text Alternative (Chapter 20)
- Figure 20.13 Text Alternative (Chapter 20)
- Figure 20.14 Text Alternative (Chapter 20)
- Figure 20.15 Text Alternative (Chapter 20)
- Figure 20.16 Text Alternative (Chapter 20)
- Figure 20.17 Text Alternative (Chapter 20)
- Figure 20.18 Text Alternative (Chapter 20)
- A Western Blot Using an Antibody Graphic Text Alternative (Chapter 20)
- DNA Strands from a Cloned Gene Graphic Text Alternative (Chapter 20)
- A Sequencing Ladder Graphic Text Alternative (Chapter 20)
- Two Sequencing Ladders Graphic Text Alternative (Chapter 20)
- DNA Sequencing Reaction Graphic Text Alternative (Chapter 20)
- Encoded by a Particular Gene Graphic Text Alternative (Chapter 20)
- Constitute Hemoglobin Graphic Text Alternative (Chapter 20)
- An Electrophoretic Mobility Shift Assay Graphic Text Alternative (Chapter 20)
- An Electrophoretic Mobility Graphic Text Alternative (Chapter 20)
- A DNAse 1 Footprinting Assay Graphic Text Alternative (Chapter 20)
- Figure 21.1 Text Alternative (Chapter 21)
- Figure 21.2 Text Alternative (Chapter 21)
- Figure 21.4 Text Alternative (Chapter 21)
- Figure 21.6 Text Alternative (Chapter 21)
- Figure 21.7 Text Alternative (Chapter 21)
- Figure 21.9 Text Alternative (Chapter 21)
- Figure 21.10 Text Alternative (Chapter 21)
- Figure 21.11 Text Alternative (Chapter 21)
- Figure 21.13b Text Alternative (Chapter 21)
- Figure 21.14a Text Alternative (Chapter 21)
- Figure 21.15 Text Alternative (Chapter 21)
- Figure 22.1 Text Alternative (Chapter 22)
- Figure 22.2 Text Alternative (Chapter 22)
- Figure 22.4 Text Alternative (Chapter 22)
- Figure 22.5 Text Alternative (Chapter 22)
- Figure 22.6 Text Alternative (Chapter 22)
- Figure 22.7 Text Alternative (Chapter 22)
- Figure 22.8 Text Alternative (Chapter 22)
- Figure 22.9 Text Alternative (Chapter 22)
- Figure 22.10 Text Alternative (Chapter 22)
- Figure 22.11 Text Alternative (Chapter 22)
- The Location of the CFTR Gene Graphic Text Alternative (Chapter 22)
- The H. Influenzae Genome Graphic Text Alternative (Chapter 22)
- Four gels Graphic Text Alternative (Chapter 22)
- A Gel Graphic Text Alternative (Chapter 22)
- Three Gels Graphic Text Alternative (Chapter 22)
- Figure 23.1 Text Alternative (Chapter 23)
- Figure 23.2 Text Alternative (Chapter 23)
- Figure 23.3 Text Alternative (Chapter 23)
- Figure 23.4 Text Alternative (Chapter 23)
- Figure 23.5 Text Alternative (Chapter 23)
- Figure 23.6 Text Alternative (Chapter 23)
- Figure 23.7 Text Alternative (Chapter 23)
- Figure 23.8 Text Alternative (Chapter 23)
- Figure 23.9 Text Alternative (Chapter 23)
- Sequence files are analyzed Graphic Text Alternative (Chapter 23)
- A multiple-sequence alignment Graphic Text Alternative (Chapter 23)
- Figure 24.2 Text Alternative (Chapter 24)
- Figure 24.3 Text Alternative (Chapter 24)
- Figure 24.4 Text Alternative (Chapter 24)
- Figure 24.5 Text Alternative (Chapter 24)
- Figure 24.6 Text Alternative (Chapter 24)
- Figure 24.7 Text Alternative (Chapter 24)
- Figure 24.8 Text Alternative (Chapter 24)
- Figure 24.9 Text Alternative (Chapter 24)
- Figure 24.10 Text Alternative (Chapter 24)
- Figure 24.11 Text Alternative (Chapter 24)
- Figure 24.12 Text Alternative (Chapter 24)
- A Punnett Square Graphic Text Alternative (Chapter 24)
- A Human Pedigree Graphic Text Alternative (Chapter 24)
- Protocol day Graphic Text Alternative (Chapter 24)
- The pedigree presented Graphic Text Alternative (Chapter 24)
- Ehler-Danlos Syndrome Graphic Text Alternative (Chapter 24)
- Hurler Syndrome Graphic Text Alternative (Chapter 24)
- Lesch-Nyhan Syndrome Graphic Text Alternative (Chapter 24)
- Sandhoff Disease Graphic Text Alternative (Chapter 24)
- Detect a Polypeptide Graphic Text Alternative (Chapter 24)
- An Experimental Assay Graphic Text Alternative (Chapter 24)
- Figure 25.1 Text Alternative (Chapter 25)
- Figure 25.2 Text Alternative (Chapter 25)
- Figure 25.3 Text Alternative (Chapter 25)
- Figure 25.4 Text Alternative (Chapter 25)
- Figure 25.5 Text Alternative (Chapter 25)
- Figure 25.6 Text Alternative (Chapter 25)
- Figure 25.7 Text Alternative (Chapter 25)
- Figure 25.8 Text Alternative (Chapter 25)
- Figure 25.9 Text Alternative (Chapter 25)
- Figure 25.10 Text Alternative (Chapter 25)
- Figure 26.1a, c Text Alternative (Chapter 26)
- Figure 26.2b, c Text Alternative (Chapter 26)
- Figure 26.3 Text Alternative (Chapter 26)
- Figure 26.5 Text Alternative (Chapter 26)
- Figure 26.6 Text Alternative (Chapter 26)
- Figure 26.9a Text Alternative (Chapter 26)
- Figure 26.10 Text Alternative (Chapter 26)
- Figure 26.11 Text Alternative (Chapter 26)
- Figure 26.12 Text Alternative (Chapter 26)
- Figure 26.13 Text Alternative (Chapter 26)
- Figure 26.15b Text Alternative (Chapter 26)
- Figure 26.16 Text Alternative (Chapter 26)
- Figure 26.17 Text Alternative (Chapter 26)
- T-cell lineages in different strains Graphic Text Alternative (Chapter 26)
- Figure 26.18 Text Alternative (Chapter 26)
- Figure 26.19 Text Alternative (Chapter 26)
- Figure 26.20 Text Alternative (Chapter 26)
- Figure 26.21 Text Alternative (Chapter 26)
- Figure 26.23 Text Alternative (Chapter 26)
- Figure 26.24 Text Alternative (Chapter 26)
- Figure 26.26 Text Alternative (Chapter 26)
- Figure 26.27 Text Alternative (Chapter 26)
- Region of the Drosophila Embryo Graphic Text Alternative (Chapter 26)
- A Hypothetical Cell Lineage Graphic Text Alternative (Chapter 26)
- Cell Lineages Graphic Text Alternative (Chapter 26)
- Northern blot Graphic Text Alternative (Chapter 26)
- Situ Hybridization Experiment Graphic Text Alternative (Chapter 26)
- Figure 27.3 Text Alternative (Chapter 27)
- Figure 27.4 Text Alternative (Chapter 27)
- Figure 27.5 Text Alternative (Chapter 27)
- Figure 27.6 Text Alternative (Chapter 27)
- Figure 27.7 Text Alternative (Chapter 27)
- Figure 27.8 Text Alternative (Chapter 27)
- Figure 27.10 Text Alternative (Chapter 27)
- Beak Depths of the Medium Ground Graphic Text Alternative (Chapter 27)
- Figure 27.11 Text Alternative (Chapter 27)
- Figure 27.13 Text Alternative (Chapter 27)
- Figure 27.15 Text Alternative (Chapter 27)
- Figure 27.16 Text Alternative (Chapter 27)
- Figure 27.17a Text Alternative (Chapter 27)
- Figure 27.18 Text Alternative (Chapter 27)
- Figure 27.19 Text Alternative (Chapter 27)
- Figure 27.20 Text Alternative (Chapter 27)
- Figure 27.21 Text Alternative (Chapter 27)
- Figure 27.22 Text Alternative (Chapter 27)
- Individual VII-1 Graphic Text Alternative (Chapter 27)
- A DNA Fingerprint Graphic Text Alternative (Chapter 27)
- Individual VI-1 Graphic Text Alternative (Chapter 27)
- A Family Pedigree Graphic Text Alternative (Chapter 27)
- Individual IV-3 Graphic Text Alternative (Chapter 27)
- Traditional DNA Fingerprints Graphic Text Alternative (Chapter 27)
- Figure 28.1 Text Alternative (Chapter 28)
- Figure 28.2 Text Alternative (Chapter 28)
- Figure 28.3b Text Alternative (Chapter 28)
- Figure 28.4 Text Alternative (Chapter 28)
- Figure 28.5 Text Alternative (Chapter 28)
- Figure 28.6 Text Alternative (Chapter 28)
- Figure 28.7b, c Text Alternative (Chapter 28)
- Figure 28.8 Text Alternative (Chapter 28)
- Figure 28.10 Text Alternative (Chapter 28)
- Figure 28.11 Text Alternative (Chapter 28)
- Figure 28.12 Text Alternative (Chapter 28)
- The Systolic Blood Pressure Graphic Text Alternative (Chapter 28)
- Figure 29.1 Text Alternative (Chapter 29)
- Figure 29.2 Text Alternative (Chapter 29)
- Figure 29.4 Text Alternative (Chapter 29)
- Figure 29.5 Text Alternative (Chapter 29)
- Figure 29.6 Text Alternative (Chapter 29)
- Figure 29.7 Text Alternative (Chapter 29)
- Figure 29.8 Text Alternative (Chapter 29)
- Figure 29.9 Text Alternative (Chapter 29)
- Figure 29.10 Text Alternative (Chapter 29)
- Figure 29.11 Text Alternative (Chapter 29)
- Figure 29.12 Text Alternative (Chapter 29)
- Figure 29.13 Text Alternative (Chapter 29)
- Figure 29.14 Text Alternative (Chapter 29)
- Figure 29.15 Text Alternative (Chapter 29)
- Figure 29.16 Text Alternative (Chapter 29)
- Figure 29.17 Text Alternative (Chapter 29)
- The Data Graphic Text Alternative (Chapter 29)
- Figure 29.18 Text Alternative (Chapter 29)
- Figure 29.19 Text Alternative (Chapter 29)
- Figure 29.20 Text Alternative (Chapter 29)
- Every learner is unique Graphic Text Alternative (FM)
- GENETIC TIPS Graphic Text Alternative (FM)
- BACKGROUND OBSERVATIONS Graphic Text Alternative (FM)
- THE HYPOTHESIS Graphic Text Alternative (FM)
- THE HYPOTHESIS OR ACHIEVING Graphic Text Alternative (FM)
- THE DATA Graphic Text Alternative (FM)
- INTERPRETING THE DATA Graphic Text Alternative (FM)
- GENES Graphic Text Alternative (FM)
- Figure A.2 Text Alternative (Appendix A)
- Figure A.3 Text Alternative (Appendix A)
- Figure A.4 Text Alternative (Appendix A)
- Figure A.5 Text Alternative (Appendix A)
- Figure A.6 Text Alternative (Appendix A)
- Figure A.7 Text Alternative (Appendix A)
- Figure A.8 Text Alternative (Appendix A)
- Figure A.9 Text Alternative (Appendix A)
- A Punnett Square Graphic Text Alternative (Appendix B)
- Female and Male Gametes Graphic Text Alternative (Appendix B)
- X-linked genes Graphic Text Alternative (Appendix B)
- In heterozygous females of fruit flies Graphic Text Alternative (Appendix B)
- A Male’s X-Linked Genes Graphic Text Alternative (Appendix B)
- The other cell will receive chromosomes Graphic Text Alternative (Appendix B)
- From Left to Right Graphic Text Alternative (Appendix B)
- The Sequence of Genes Graphic Text Alternative (Appendix B)
- Transposon Graphic Text Alternative (Appendix B)
- An Unusual Strand of DNA Graphic Text Alternative (Appendix B)
- A Northern Blot with 5 Lanes Graphic Text Alternative (Appendix B)
- A Single mRNA Graphic Text Alternative (Appendix B)
- Two Likely Scenarios Graphic Text Alternative (Appendix B)
- Chromosome Graphic Text Alternative (Appendix B)
- Dominant Trait Graphic Text Alternative (Appendix B)
UM RAFBÆKUR Á HEIMKAUP.IS
Bókahillan þín er þitt svæði og þar eru bækurnar þínar geymdar. Þú kemst í bókahilluna þína hvar og hvenær sem er í tölvu eða snjalltæki. Einfalt og þægilegt!Rafbók til eignar
Rafbók til eignar þarf að hlaða niður á þau tæki sem þú vilt nota innan eins árs frá því bókin er keypt.
Þú kemst í bækurnar hvar sem er
Þú getur nálgast allar raf(skóla)bækurnar þínar á einu augabragði, hvar og hvenær sem er í bókahillunni þinni. Engin taska, enginn kyndill og ekkert vesen (hvað þá yfirvigt).
Auðvelt að fletta og leita
Þú getur flakkað milli síðna og kafla eins og þér hentar best og farið beint í ákveðna kafla úr efnisyfirlitinu. Í leitinni finnur þú orð, kafla eða síður í einum smelli.
Glósur og yfirstrikanir
Þú getur auðkennt textabrot með mismunandi litum og skrifað glósur að vild í rafbókina. Þú getur jafnvel séð glósur og yfirstrikanir hjá bekkjarsystkinum og kennara ef þeir leyfa það. Allt á einum stað.
Hvað viltu sjá? / Þú ræður hvernig síðan lítur út
Þú lagar síðuna að þínum þörfum. Stækkaðu eða minnkaðu myndir og texta með multi-level zoom til að sjá síðuna eins og þér hentar best í þínu námi.
Fleiri góðir kostir
- Þú getur prentað síður úr bókinni (innan þeirra marka sem útgefandinn setur)
- Möguleiki á tengingu við annað stafrænt og gagnvirkt efni, svo sem myndbönd eða spurningar úr efninu
- Auðvelt að afrita og líma efni/texta fyrir t.d. heimaverkefni eða ritgerðir
- Styður tækni sem hjálpar nemendum með sjón- eða heyrnarskerðingu
- Gerð : 208
- Höfundur : 15114
- Útgáfuár : 2023
- Leyfi : 380