Hydrology and the Management of Watersheds
10.890 kr.
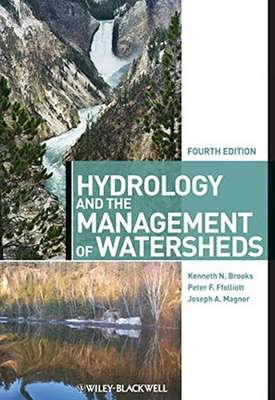
Námskeið
- Vatnshagur.
Ensk lýsing:
Comprehensive introductory reference on hydrology and watershed management principles, methods and applicationsLýsing:
Comprehensive introductory reference on hydrology and watershed management principles, methods and applicationsAnnað
- Höfundur: Kenneth N. Brooks, Peter F. Ffolliott, Joseph A. Magner
- Útgáfa:4
- Útgáfudagur: 2012-12-14
- Engar takmarkanir á útprentun
- Engar takmarkanir afritun
- Format:ePub
- ISBN 13: 9781119104292
- Print ISBN: 9780470963050
- ISBN 10: 1119104297
Efnisyfirlit
- Front Matter
- DEDICATION
- PREFACE
- DEFINITION OF TERMS
- PART 1 Watersheds, Hydrologic Processes, and Pathways
- Watersheds, Hydrologic Processes, and Pathways
- Photo 1. Measuring groundwater levels in a forested wetland with a pressure transducer in a shallow well
- Photo 2. Forested headwater watersheds are the source of most of the streamflow in the United States as depicted in this scene in the Northern Cascades of Washington
- Photo 3. Students measuring streamflow with a current meter
- CHAPTER 1 Introduction
- Overview
- Box 1.1 A Historical Look at Hydrology, Water, Watershed Management, and People
- Watersheds
- Watersheds and Stream Orders
- Figure 1.1. Stream order system by Horton (1945) as modified by Strahler (1964)
- A Geomorphologic Perspective
- Figure 1.2. Rivers generally flow from an upper, high-gradient erosion zone through a transition zone to a low-gradient deposition zone
- Watershed Assessments
- Watersheds and Stream Orders
- Integrated Watershed Management
- Sustainable Use and Development of Natural Resources
- Land and Water Scarcity
- Land Scarcity
- Water Scarcity
- Coping with Hydrometeorological Extremes
- Table 1.1. The role of watershed management in developing solutions to natural resource problems
- Box 1.2 Water – The Top Global Environmental Concern?
- Box 1.3 Effects of Altering Natural Ecosystems on Flooding: Examples in the Upper Midwest, United States
- Land and Water Scarcity
- Overview
- Watersheds, Ecosystem Management, and Cumulative Effects
- Reconciling Watershed and Political Boundaries
- Box 1.4 Minnesota's Major Watershed Restoration and Protection Strategy
- Box 1.5 The Nile River Basin: A Case for Watershed Management (Baecher et al., 2000)
- Summary and Learning Points
- References
- Webliography
- Watersheds, Hydrologic Processes, and Pathways
- CHAPTER 2 Hydrologic Cycle and the Water Budget
- Introduction
- Properties of Water
- Figure 2.1. Water droplet on hydrophobic soil illustrates the cohesive property of water molecules
- Importance of Polarity
- Table 2.1. The unique water molecule properties of water at normal terrestrial temperatures (Maidment 1993 and others)
- State of Water
- The Hydrologic Cycle
- Hydrologic Processes
- Figure 2.2. The hydrologic cycle
- Figure 2.3. Distribution of freshwater on earth from van der Leeden et al. (1990)
- Water Budget
- Soil Moisture and the Water Budget
- Figure 2.4. The hydrologic cycle consists of a system of water storage compartments and the solid, liquid, or gaseous flows of water within and between the storage points
- Figure 2.5. Typical water-holding characteristics of soils of different textures. C, clay; F, fine; H, heavy; L, loam; Lt, light; S, sand; St, silt; and Sy, sandy
- Box 2.1 Steps in Determining Soil Water Content and Change in Soil Moisture for Water Budget Application
- Figure 2.6. Soil water content in a column of soil can be expressed as an equivalent depth of water; in this case, the water content in the soil is equivalent to 15-cm depth
- Applying the Water Budget Approach
- Figure 2.7. Hypothetical fluctuation of soil moisture on an annual basis
- Soil Moisture and the Water Budget
- Hydrologic Processes
- Radiation
- Table 2.2. Albedos of natural terrestrial surfaces
- Energy Budget
- Table 2.3. Energy budget measurements at Akron, CO, USA, for three different conditions
- Box 2.2 Energy Budget Components for Oasis Conditions at Aspendale, Australia (Adapted from Penman et al., 1967)
- Box 2.3 Energy and Water Budgets in a Changing Climate
- Energy and the Flow of Liquid Water
- Figure 2.8. Matric potential (ψm) and pressure potential (ψp) of a dry soil (A), moist soil (B), at the water table (WT), and in a saturated soil (C) measured with a water manometer or tensiometer.
- Units Commonly Used to Express Water Potential
- Figure 2.9. Water flow through soil moves from a higher water potential (ψ) or wetter soil to a lower water potential or drier soil. As the soil dries at the soil surface (or at the soil–root interface), the resistance to water flow (rs) increases as a result of the more tortuous pathway of flow through the thinner water films surrounding soil particles
- Introduction
- Precipitation Process
- Box 3.1 Forms of Precipitation
- Figure 3.1. The relationship of saturation vapor pressure and air temperature. A parcel of unsaturated air (A) must be cooled (A to C) or moisture must be added (A to B) before saturation occurs
- Figure 3.2. Mechanisms causing air masses to lift in elevation and cool and result in precipitation
- Rainfall
- Methods of Rainfall Measurement
- Figure 3.3. Types of rain gauges. (a) Cutaway of the standard (Weather Service) gauge. (b) A weighing-type recording gauge with its cover removed to show the spring housing, recording pen, and storage bucket
- Figure 3.4. Tipping-bucket gauge
- Figure 3.5. Proper siting of a rain gauge with respect to the nearest object
- Table 3.1. Effect of wind velocities on the catch of precipitation by standard rainfall gauges
- Number of Gauges Required
- Calculating Mean Rainfall on a Watershed
- Arithmetic Mean
- Figure 3.6. Arithmetic mean, Thiessen polygon, and isohyetal methods of calculating the mean rainfall on a watershed
- Thiessen Polygon Method
- Isohyetal Method
- Other Methods
- Arithmetic Mean
- Errors Associated with Rainfall Measurement
- Analysis of Rainfall Measurements
- Estimating Missing Data
- Double Mass Analysis
- Frequency Analysis
- Figure 3.7. A double mass plot of the annual rainfall at station E relative to the average annual rainfall at stations H and I
- Figure 3.8. Frequency curve of the daily rainfall recorded for a single station
- Figure 3.9. Intensity duration frequency curves for the Midwestern United States
- Figure 3.10. Relationship between maximum rainfall amounts for specified durations and area
- Depth–Area–Duration Analysis
- Methods of Rainfall Measurement
- Box 3.2 Terminology Used in Snow Hydrology
- Methods of Snowfall Measurement
- Snow Surveys
- Box 3.3 Forecasting Future Water Supplies from a Snow Survey
- Remote Sensing Methods
- Snow Surveys
- Analysis of Snowfall Measurements
- Snow Accumulation and Metamorphism
- Cold Content.
- Liquid-Water-Holding Capacity.
- Box 3.4 Cold Content Calculation for a Snowpack of Depth = 100 cm, Snow Density = 0.1 g/cm3, Temperature Deficit (Ts) = −10°C
- Total Retention Storage.
- Snow Accumulation and Metamorphism
- Energy Budget and Snowmelt Relationships
- Generalized Snowmelt Equations
- Solar Radiation.
- Box 3.5 Determining Snow Thermal Quality by the Calorimeter Method (Snow Depth = 60 cm, Volume of Snow Sample Taken = 15,000 cm3, and Weight of Sample = 2000 g) (modified from Hewlett 1982)
- Figure 3.11. Relationship between solar radiation and cloud height and cloud cover
- Figure 3.12. Relationship between coniferous forest canopy density and transmission of solar radiation
- Longwave Radiation.
- Net Radiation.
- Figure 3.13. Net radiation of a snowpack in relation to a forest canopy cover
- Convection–Condensation Melt.
- Rain Melt.
- Conduction Melt.
- Combined Snowmelt Equations.
- Solar Radiation.
- Temperature Index (Degree-Day) Method
- Figure 3.14. Temperature index relationships for maximum daily air temperatures
- Table 3.2. Base temperature and melt-rate indices for three watersheds in the western United States
- Introduction
- The Evaporation Process
- Evaporation from Water Bodies
- Table 4.1. Evaporation from selected lakes and reservoirs in the United States and Australia
- Evaporation from Soil Surfaces
- Interception
- Figure 4.1. Components of interception. Ic, canopy interception loss; Il, litter interception; Pg, gross precipitation; Pn, net precipitation; Sf, stem flow; Th, throughfall
- Components of Interception
- Throughfall
- Box 4.1 Throughfall Relationships for Different Vegetative Canopies
- Stemflow
- Throughfall
- Interception Process
- Box 4.2 Deposition of Intercepted Snowfall
- Hydrologic Importance of Interception
- Table 4.2. Interception of forest types
- Table 4.3. Interception storage for red pine stands at the Cloquet Forestry Center, Minnesota
- Table 4.4. Simulated interception components for the growing season (June, July, and August) for four red pine stands at Cloquet Forest Center, Minnesota, as described in Table 4.3
- Figure 4.2. A representation of soil–plant–atmospheric resistances to water flow (a) and corresponding flow through the roots and leaves (b) The flow of water can be described by V = Δ ψ/r, that is analogous to Ohm's law
- Measurement of Transpiration
- Figure 4.3. Relation between stomatal width and transpiration in still-air and windy conditions
- Potted Plants and Lysimeters
- Figure 4.4. Changes in water potential (ψ) of soil, root, and leaf as transpiration occurs beginning with a soil near field capacity and proceeding until the permanent wilting point (−15 bars) is reached
- Measurement of Sap Flow in Woody Plants
- Figure 4.5. Sap flow velocity method using heat as a tracer; heat dissipation in stem on the left indicates no transpiration while the stem on the right indicates transpiration
- Tent Method
- Other Methods
- Box 4.3 Annual Transpiration of Oak Trees in Southeastern Arizona (Ffolliott et al., 2008)
- Figure 4.6. Triple-inlet evaporation tent. 1, inlet; 2, squirrel-cage blower; 3, inlet humidity thermometer; 4, perforated polyvinyl curtain; 5, outlet; and 6, outlet humidity thermometer
- Table 4.5. Maximum daily transpiration rates for selected tree species
- Figure 4.7. Effects of changing vegetative cover on transpiration and total ET. (a) Soil water depletion (cross-hatched areas) of a bare soil, a grassland, and a mature forest (vertical scale exaggerated). (b) The associated accumulated evaporation–evapotranspiration for bare soil conditions
- Table 4.6. Controls of ET under different climatic conditions and types of vegetation
- Evapotranspiration/Potential Evapotranspiration Approach
- Box 4.4 The Paired-Watershed Method as a Means of Estimating the Effects of Timber Harvesting on Streamflow
- Figure 4.8. Actual evapotranspiration (AET) to potential evapotranspiration (PET) ratio as a function of water conditions for an old-growth forest (A), intermediate forest cover condition (B), and open or clearcut condition (C). FC, field capacity
- Water Budget Approach
- Figure 4.9. Effects of time and tree species on soil water deficit. τ, limiting soil water deficit with time for the tree species; tc, time (in years) when soil water begins to limit ET; tr, time (in years) when the hydrologic effect of clearcutting becomes negligible
- Box 4.5 Water Budget Exercise for a Forest-Covered Watershed, Near Chiang Mai, Thailand
- Introduction
- Infiltration
- Figure 5.1. Infiltration capacity curves for soils (A) governed by soil textural porosity and (B) governed by macropores that have variable continuity with depth
- Figure 5.2. Relationship between rainfall rate and infiltration rate resulting in surface runoff or ponding
- Infiltration Capacity
- Figure 5.3. Effects of surface roughness and surface sealing on infiltration of a soil with a macropore and micropore systems
- Measurement of Infiltration
- Infiltration Equations
- Box 5.1 Infiltration Equations
- Figure 5.4. Darcy's laboratory method of determining the hydraulic conductivity (kv) of a soil for a given head of water (ΔH) and length of soil column (L) and measuring the quantity of flow per unit time (Q) through area (A)
- Land-use Impacts on Infiltration
- Table 5.1. Net (or final) infiltration rates for unfrozen soils
- Water-Repellent Soils
- Table 5.2. Comparisons of soils and infiltration relationships (double-ring infiltrometers) for three land-use conditions in northern Morocco
- Figure 5.5. Effect of water-repellent layer on infiltration and surface runoff.
- Figure 5.6. Infiltration rates of water-repellent soil (shown in Figure 5.5) and wettable soil
- Box 5.2 The Role of Fire and Soil Heating in Intensifying Water Repellency (from DeBano, 2000b)
- Soil Frost
- Figure 5.7. Effects of soil frost on infiltration rates
- Figure 5.8. Depth of soil frost during midwinter (January to mid-March) on loamy soils in northern Minnesota under different cover types; the vertical scale is exaggerated
- Groundwater Recharge
- Figure 5.9. Types of groundwater recharge found in varying climatic and geologic settings.
- Groundwater Recharge Zones and Land Use
- Methods of Estimating Groundwater Recharge
- Box 5.3 Exploring a Watershed-based Approach to Groundwater Recharge (Used with Permission from Peterson, 2011)
- Box 5.3. Minimum groundwater recharge rates predicted by the WCA for the Sauk River, Minnesota, from 1955–1978
- Box 5.3 Exploring a Watershed-based Approach to Groundwater Recharge (Used with Permission from Peterson, 2011)
- Baseflow
- Figure 5.10. Relationship between pathways of water flow from a watersheds and the resultant streamflow hydrograph. A, channel interception; B, surface runoff; C, subsurface flow or interflow; D, groundwater or base flow; Q, streamflow discharge
- Stormflow.
- Box 5.4 Stormflow Response of Hillslopes and Small Watersheds (from Beasley, 1976)
- Figure 5.11. Schematic of the variable source area of stormflow and the relationship between overland flow of water and the zone of no infiltration. The small arrows on the hydrographs indicate streamflow-response changes as the variable source area expands
- Introduction
- Measurement of Streamflow
- Measuring Discharge
- Figure 6.1. Measurements of stream channel cross sections and velocities needed to estimate the mean velocity of a stream
- Figure 6.2. Example of a rating curve of streamflow discharge in relation to depth measurements. A logarithmic relationship is shown in the figure because of the range of measurements obtained
- Precalibrated Structures for Streamflow Measurement
- Weirs
- Figure 6.3. A schematic illustration of a weir with a V-shaped notch on the weir blade
- Flumes
- Figure 6.4. A broad-crested trapezoidal flume for measuring open channel streamflow
- Considerations for Using Precalibrated Structures
- Weirs
- Monitoring Streamflow in the United States
- Obtaining Discharge Information on Ungauged Streams
- Figure 6.5. Stream channel section showing the slope or gradient of the streambed, wetted perimeter WP (a, b, c, d), and cross-sectional area A
- Measuring Discharge
- Table 6.1. Examples of Manning's roughness coefficient n
- Figure 6.6. Evidence of high watermarks in a stream for estimating the depth of flow
- Direct Transfer of Streamflow Information
- Table 6.2. Values of runoff coefficients (C) for the rational method
- Estimating Peak Discharge
- Box 6.1 Example of Applying the Rational Method to Determine the Size of a Culvert
- Stormflow Response
- Stormflow Response Factor
- Box 6.2 Separating Baseflow from Total Streamflow Resulting from a Storm Eevent
- Figure for Box 6.2. Methods of separating baseflow from stormflow; I and II are different approaches.
- Box 6.2 Separating Baseflow from Total Streamflow Resulting from a Storm Eevent
- Stormflow Response Factor
- Unit Hydrograph
- Box 6.3 Applying the Response Factor Method to Estimate Storage Requirements
- Development of a Unit Hydrograph.
- Figure 6.7. Development of a UHG for an isolated storm
- Table 6.3. Application of a 1-h UHG to a storm of 2 h of effective precipitation
- Application of a Unit Hydrograph.
- Figure 6.8. S-curve method of converting a UHG of one duration of P (mm/h) effective rainfall to another (Ti)
- Loss Rate Analysis.
- Synthetic Unit Hydrographs.
- Figure 6.9. SCS triangular hydrograph
- Table 6.4. Runoff CNs for hydrologic soil-cover complexes for AMC II
- Figure 6.10. Rainfall-stormflow relationships for CNs
- Box 6.4 Application of the SCS Triangular Hydrograph and CN Method
- Figure 6.11. Example of reservoir-routing method for streamflow routing
- Figure 6.12. Annual- and partial-duration series approaches to peak flood analysis
- Graphical-Frequency Analysis
- Analytical-Frequency Analysis
- Frequency Analysis on Ungauged Watersheds
- Figure 6.13. Analytical-frequency curve with graphical plotting positions of annual peak discharge for the Little North Santiam River near Mehama, Oregon (1932–1950)
- Comments on Frequency Analysis
- Introduction
- Groundwater
- Basic Concepts
- Figure 7.1. Groundwater characteristics and water table changes from a wet to a dry season
- Storage and Movement of Groundwater
- Unconfined and Confined Aquifers
- Box 7.1 Connate Water in the Red River Valley of the North, USA (Magner et al., 2001)
- Figure 7.2. Artesian (confined) aquifer and recharge area with a perched water table above an impermeable layer
- Aquifer Characteristics
- Figure 7.3. Pressure head (hp), elevation head (z), and total hydraulic head (ht) of water. Elevation head (z) is the distance from an arbitrary but stable reference datum to a point (a) where the pressure head (hp) is measured
- Box 7.2 Application of Darcy's Law
- Table 7.1. Examples of hydraulic conductivities for unconsolidated sediments (pure water, 15.6°C)
- Unconfined and Confined Aquifers
- Basic Concepts
- Groundwater Development
- Table 7.2. Water-bearing and yield characteristics of some common aquifers
- Wells
- Box 7.3 Construction of Wells with Drilling Rigs
- Management Considerations
- Figure 7.4. Cones of depression
- Groundwater Recharge Considerations
- Effects of Vegetation on Groundwater
- Box 7.4 Determining the Source of Groundwater
- Table 7.3. Annual estimates of evapotranspiration from phreatophytes in the southwestern USA
- Linkage to Streams and Riparian Areas
- Figure 7.5. Water in the hyporheic zone is exchanged above with surface water, below with groundwater, and laterally with riparian corridor and alluvial aquifers
- Box 7.5 Sources of Baseflow to a Flowing River: One Example (Wirt and Hjalmarson, 2000)
- Linkage to Lakes
- Figure 7.6. Typical hydraulic conditions between inflowing groundwater and a lake show how flow lines are closer to each other at shoreline and then become more distant further into the lake bed
- Figure 7.7. An example of the effect of transpiration on water movement; note the drop in the water table directly below the root zone during the growing season and the potential to pull lake water toward the root zone
- Box 7.6 Determining Lake–Groundwater Exchanges (Winter et al., 1998)
- Linkage to Wetlands
- Box 7.7 Examples of Wetland Types
- Physical, Chemical, and Biological Linkages of Water Flow
- Photo 4. Unstable stream channels result in bluff erosion as depicted in the stream in southern Minnesota, USA
- Photo 5. Soil mass erosion can provide sediment to streams as depicted in this scene in southwestern Montana, USA
- CHAPTER 8 Soil Erosion Processes and Control
- Introduction
- Surface Soil Erosion
- Figure 8.1. Major active and passive factors that affect erosion and sediment transport from a land surface
- Wind Erosion
- Surface Erosion by Water
- Table 8.1. Kinetic energy (Ke) associated with different intensities of rainfall and illustration of soil displacement due to rainfall impact
- Figure 8.2. Surface soil erosion as a result of raindrop impact and turbulent surface runoff
- Measurement of Surface Erosion
- Erosion Plots.
- Figure 8.3. A plot to measure runoff and soil erosion
- Erosion Stakes.
- Natural Landscape Features.
- Erosion Plots.
- Prediction of Soil Loss
- Universal Soil Loss Equation.
- Rainfall Erosivity Factor.
- Soil Erodibility Factor.
- Figure 8.4. Nomograph for determining the soil erodibility factor (K) in English units
- Slope and Gradient Factor.
- Cropping Management Factor.
- Erosion Control Practice Factor.
- Modified USLE (MSLE).
- Table 8.2. Application of USLE and MULSE under a variety of conditions
- Figure 8.5. Relationships of forest canopy cover (a), ground cover (b), and fine roots in the topsoil (c) used to determine subfactors I, II, and III, respectively, for the VM factor
- Figure 8.6. Relationship between ground cover conditions and the VM factor for the Modified Soil Loss Equation
- Soil Loss Tolerance.
- Box 8.1 Application of the Modified USLE (MSLE)
- Table 8.3. C or VM factors for permanent pasture, rangeland, idle land, and grazed woodland
- Revised Universal Soil Loss Equation.
- Water Erosion Prediction Project Model.
- Box 8.2 Application of Modified USLE and the GeoWEPP Model for Predicting Soil Erosion from Roads and Trails (Renschler and Flanagan, 2008)
- Preventing and Controlling Surface Erosion
- Box 8.3 Surface Erosion in Dryland Africa Increases Drastically after Removal of Vegetative Cover (Harrison, 1987)
- Table 8.4. Guidelines for preventing water and wind erosion
- Surface Erosion Control in Forest Lands
- Figure 8.7. Relationship between erosion rates and annual precipitation for vegetation types and watershed cover conditions
- Surface Erosion Control on Rangelands
- Mechanical Methods of Controlling Surface Soil Erosion
- Gully Erosion Process
- Figure 8.8. Illustration of gully formation and headwall retreat over time
- Box 8.4 Use of Field Surveys and Aerial Photo Interpretation to Estimate Gully Erosion and Sources of Sediment (from Stromquist et al., 1985
- Gully and sheet erosion over time on a 6.15 km2 in southeastern Lesotho.
- Vegetation Establishment
- Mechanical Structures
- Check Dams
- Figure 8.9. Diagram of placement of check dams: Sp, spacing; θ, angle of gully gradient
- Headcut Control
- Vegetation-Lined Waterways
- Figure 8.10. Illustration of soil mass movements
- Box 8.5 Soil Mass Movement and Debris Flows in Taiwan
- Table 8.5. Classification of hillslope failures
- Figure 8.11. Simplified diagram of forces acting on a soil mass on a slope
- Factors Affecting Hillslope Stability
- Evaluating the Stability of Hillslopes
- Reducing Impacts of Soil Mass Movement
- Cumulative Effects of Soil Mass Movement
- Box 8.6 Factors to Consider When Making Hazard Assessments of Hillslope Failure (from Swanston and Swanson, 1980)
- Figure 8.12. Hypothetical relationship of root-strength deterioration after timber harvesting and root-strength improvement with regenerating forest
- Introduction
- Sediment Supply and Transport
- Sources of Sediment
- Energy Relationships of Streams
- Subcritical and Supercritical Flow
- Table 9.1. Terminology used in describing channel dynamics and processes
- Laminar and Turbulent Flow
- Subcritical and Supercritical Flow
- Sediment Movement
- Types of Sediment Transported in a Stream
- Figure 9.1. Transportation of particles of sediment in running water
- Suspended Load.
- Figure 9.2. Example of the relationship between streamflow discharge and suspended sediment for a small stream in northeastern Minnesota. a: the relationship for several storm events; b: a single storm event (included in A)
- Bed Load.
- Box 9.1 Sediment rating curves for watersheds of Arizona
- Relationship between streamflow discharge and suspended sediment from a clearcut ponderosa pine watershed in north-central Arizona
- Box 9.1 Sediment rating curves for watersheds of Arizona
- Types of Sediment Transported in a Stream
- Degradation and Aggradation
- Figure 9.3. Relationships involved in maintaining a stable channel balance
- Figure 9.4. Rate of sedimentation as affected by transport capability and supply rate for different-sized particles for a particular stream and flow condition
- Dynamic Equilibrium
- Suspended Sediment
- Bed Load
- Total Sediment
- Figure 9.5. A sediment splitter diverts progressively smaller fractions of streamflow to a collection tank to sample suspended sediment flowing through a rectangular weir in north-central Arizona. By also collecting bedload materials that settle in the catchment basin upstream of the weir total sediment yields can be measured from a watershed
- Table 9.2. Average annual sediment yield from selected river basins that are among the 21 largest sediment yielding rivers in the world
- Sediment Budgets
- Box 9.2 A comparison of sediment yields in two large river basins
- Table 9.3. Sediment yield from small forested watersheds and larger watersheds of mixed land use in the United States
- Figure 9.6. Sediment delivery ratio determined from watershed size
- Sediment Delivery Ratio
- Box 9.3 Cumulative watershed effects on stream sediment: two examples in the United States
- Introduction
- Fluvial Geomorphology
- Rivers, Floods, and Floodplains
- Bankfull Stage
- Figure 10.1. Landforms in a river valley and flow conditions in a channel for bankfull and the 50-year recurrence interval (RI) flood
- Figure 10.2. Effective discharge is determined as the maximum product of sediment transport rate and the frequency of occurrence of streamflow discharge
- The Floodplain
- Figure 10.3. A large rainstorm event in August 18–19, 2007, produced nearly one-half of the annual precipitation within the Garvin Brook watershed, in southeastern Minnesota, USA. While this was an infrequent event (500-year), large boulders and cobbles in the stream channel and floodplain were redistributed by extreme boundary shear and a new channel was cut through a playground at Farmers County Park
- Valley Classification Systems
- Gibling's Classification System
- Rosgen's Classification System
- Stream Channel Stability
- Table 10.1. Valley types used in the geomorphic characterization and associated stream types (derived from Rosgen, 1996, 2006)
- Figure 10.4. Channel Evolution Model moves from stable to degraded to aggregated at a new base elevation. Height h influences potential stream energy
- Schumm's Evolution Model
- Bank-Height Ratio
- Pfankuch's Procedure for Evaluating Stream Channel Stability
- Box 10.1 Channel Instability: Loess Area of the Midwestern USA (Simon and Rinaldi, 2000)
- Figure 10.5. Stream channel and bank locations used in the Pfankuch (1975) method of streambank stability rating
- Box 10.1 Channel Instability: Loess Area of the Midwestern USA (Simon and Rinaldi, 2000)
- Table 10.2. Stream reach stability evaluation
- Watershed Assessment of River Stability and Sediment Supply
- Table 10.3. Form for applying the Minnesota Agricultural Ditch Reach Assessment for Stability (MADRAS) system (Magner et al., 2012)
- Figure 10.6. Flowchart 4-1 FROM WARSSS illustrating sequential steps taken in the RLA selection process for subwatersheds and reaches that required further investigation involving field data collection
- Table 10.4. Terminology used in describing stream morphology and classification
- Rosgen's Stream Classification
- Table 10.5. Levels of the Rosgen Stream Classification system
- Figure 10.7. Major stream types with their cross-sectional configurations and physical characteristics
- Continuum Concept
- Figure 10.8. Classification system for natural rivers
- Figure 10.9. Example of evolutionary adjustments in stream channels
- Stream Channel Succession
- Interpretations of Stream Classification
- Figure 10.10. Comparison of the Channel Evolution Model and the corresponding four Rosgen stream types
- Box 10.2 Management Interpretations of Stream Types Classified by Rosgen (1994)
- Introduction
- Chemistry of Precipitation
- Acid Precipitation and Atmospheric Chemical Constituents
- Box 11.1 Sources of Contaminants in Snowpacks – An Example in Montana, United States (Pagenkopf, 1983)
- Atmospheric Deposition of Mercury
- Acid Precipitation and Atmospheric Chemical Constituents
- Physical Characteristics of Surface Water
- Suspended Sediment
- Sources
- Nutrient and Heavy-Metal Transport Capacities of Sediment
- Thermal Pollution
- Table 11.1. Relationship between the saturated solubility of oxygen in water and water temperature
- Dissolved Oxygen
- Figure 11.1. Measurement of DO with a multiparameter sonde
- Figure 11.2. A BOD curve illustrating the carbonaceous demand phase and nitrification phase
- Biochemical and Chemical Oxygen Demands
- Table 11.2. Examples of biochemical oxygen demand (BOD) values for different conditions
- Table 11.3. Examples of end products from organic loading in water bodies under aerobic and anaerobic conditions
- Figure 11.3. Effects of disposal of raw sewage in a stream on the DO and BOD for stream water, either in time or downstream. The effects of reaeration rate and the diurnal characteristics of DO are also shown
- Field-Measured Indicators and Other Physical Characteristics of Water Quality
- pH
- Figure 11.4. The relationship of accelerated bacterial growth to changes in DO and BOD due to disposal of raw sewage in a stream
- Figure 11.5. Effects of sewage disposal on species composition and populations of higher aquatic life-forms in a stream
- Acidity
- Alkalinity and Partial Pressure of CO2 Gas
- Specific Conductance
- Box 11.2 Water-Quality Influences from Row-Crop Agriculture in Southern Minnesota
- Turbidity
- pH
- Suspended Sediment
- Sources of Nutrients
- Nitrogen
- Table 11.4. Mean concentrations of nitrogen and phosphorus for different land uses in the eastern United States
- Phosphorus
- Calcium
- Magnesium
- Sodium
- Figure 11.6. Concentrations of Cl are plotted against Br in Pope County, Minnesota
- Chloride
- Potassium
- Manganese
- Sulfur
- Oxidation Reduction Potential
- Figure 11.7. Aerobic conditions as measured by Eh in millivolts (mV), plot well above zero in the +300- + 400 mV range. Suboxic waters plot above zero but below + 300 mV and represent a transitional state between oxic and reduced waters. Anaerobic or reduced waters plot near and below zero and have very unique mV conditions associated with specific species
- Pesticides and Fertilizers
- Table 11.5. Values of 48-hour median tolerance (TLM) for selected pesticides and aquatic organisms
- Nitrogen
- Bacteria
- Protozoa
- Aquatic Biota as Indicators of Surface-Water Quality
- Box 11.3 Indicating Water Quality by the Presence of Macroinvertebrates: An Index
- Box 11.4 Effects of Surface Land Use on Water Quality in Karst Aquifers
- Integrated Watershed Management
- Photo 6. The Rodeo-Chediski fire in Arizona, USA, burned more than 189,000 ha of mostly ponderosa pine forest in 2002, resulting in serious soil and water impacts
- Photo 7. Urbanization creates more surface runoff and compounds localized flooding – a 2011 scene of the Mississippi River at flood stage in St. Paul, Minnesota, USA
- CHAPTER 12 Managing Wildland Watersheds
- Introduction
- Forests
- Forest Management and Water Yield
- Figure 12.1. Streamflow from snowmelt runoff contributes much of the water originating on watersheds in the forests of the western region of the USA
- Table 12.1. Increases in water yield associated with reductions in vegetative cover for noncloud forest or coastal forest conditions
- Timber-Harvesting Effects
- Humid Temperate Regions.
- Figure 12.2. Hypothetical reduction in transpiration as a function of uniform removal (thinning) of trees and shrubs by thinning in conditions that vary from unlimited soil-water availability to plants (shaded area tending toward broken line) to limited soil-water availability (shaded portion tending toward solid line) as modified from Figure 4 in Hibbert (1979)
- Figure 12.3. Clearcuts in ponderosa pine forests of Arizona, USA, have been shown to increase water yields
- Forested Uplands in Snow-Dominated Regimes.
- Box 12.1 Effects of Strip-Cutting Forests on Snowpacks and Water Yield
- Figure. Effects of strip cutting a ponderosa pine forest in Arizona, USA, on wind patterns and snow deposition
- Box 12.1 Effects of Strip-Cutting Forests on Snowpacks and Water Yield
- Humid Temperate Regions.
- Tropical Regions.
- Cloud Forests.
- Effects of Fire
- Figure 12.4. Annual streamflow discharges from a burned watershed in the Cascade Range of eastern Washington, USA, before and after a wildfire in relation to annual streamflow discharge from a control
- Effects of Insects and Disease
- Estimating Changes in Water Yields
- Regional Relationships.
- Figure 12.5. Relationship between net and gross annual precipitation for aspen and red pine
- A Water-Budget Approach.
- Applications of Hydrologic Computer-Simulation Models.
- Box 12.2 Application of a Water-Budget Method to Estimate Changes in Water Yield due to Clearcutting a Mature Hardwood Deciduous Forest
- Table 12.2. Water budget for a hardwood-covered watershed before clearcutting
- Table 12.3. Water budget for a clearcut hardwood forest
- Regional Relationships.
- Forest Management and Water Yield
- Box 12.3 Water Resource Problems Resulting from Vegetative Changes (Drysdale, 1981)
- Effects on Stormflow
- Forest Harvesting – Rainfall Regimes.
- Forest Harvesting – Snowmelt Regimes.
- Table 12.4. Changes in rainfall-produced stormflow following forest cover removal
- Figure 12.6. Factors that affect snowmelt and runoff from a snowpack
- Figure 12.7. Relation between the portion of a watershed clearcut and the change in annual snowmelt peak discharge compared to a mature stand of hardwoods in Minnesota
- Table 12.5. Effect of wildfire on annual peak flows
- Effects of Wildfire.
- Figure 12.8. Streamflow discharge peak-flow stage shortly after cessation of the Rodeo-Chediski Wildfire in a ponderosa pine watershed in Arizona; prefire peak-discharge stage noted
- Sediment.
- Turbidity.
- Water Temperature.
- Dissolved-Oxygen Levels.
- Nutrients.
- Box 12.4 Effects of Clearcutting Trees and Site Preparation on Nutrient Export from Small Watersheds in Southeastern USA
- Transport of Nutrients and Heavy Metals in Sediment.
- Pesticides and Fertilizers.
- Fire Retardants.
- Sediment and Roads.
- Table 12.6. Pollutants observed in runoff from road surfaces
- Chemical Inputs from Roads.
- Figure 12.9. Pinyon-juniper woodlands represent the most extensive woodland type in the western USA
- Water Yield
- Woodlands and Streamflow Regimen
- Water Quality
- Vegetative Manipulations and Water Yield
- Figure 12.10. Water yields increased following a wildfire and application of herbicides on the two watersheds on the lower elevations in a chaparral community in the southwestern USA
- Stormflow Response to Management
- Figure 12.11. Annual streamflow discharges increased with increasing annual precipitation on 11 watersheds in the chaparral communities of central Arizona following wildfire and applications of herbicides to control shrub regrowth
- Water Quality
- Sediment Yields
- Chemical Characteristics
- Biological Characteristics
- Losses of Water En Route Downstream
- Upstream–Downstream Flooding Relationships
- Figure 12.12. Changes in the magnitude and recurrence interval of annual peak flows following aspen clearcutting in northern Minnesota
- Linkages to Reservoirs
- Figure 12.13. Effects of forest removal on upstream and downstream stormflow hydrographs where desynchronization of stormflow hydrographs occur
- Storage Requirements
- General Operating Procedures
- Figure 12.14. Storage allocation for a multipurpose reservoir with associated sedimentation
- Water Yield and Streamflow Regimen
- Water Quality
- Assessing Environmental Flows
- Introduction
- Riparian Communities
- Figure 13.1. Riparian ecosystems are most often associated with the presence of vegetation that requires large amounts of free or unbound water. The ecosystem illustrated in this figure is that in a dryland environment
- Characteristics of a Healthy Riparian Community
- Figure 13.2. A healthy riparian community is able to maintain an equilibrium between the streamflow forces acting to produce change and the resistance of vegetative, geomorphic, and structural features to the change. Riparian area in Honduras
- Riparian–Watershed Linkages
- Box 13.1 Characteristics of Watershed Condition
- Riparian Management
- Sustaining Buffer Strips
- Figure 13.3. A schematic of how dissolved constituents and entrained soil particles move through a riparian buffer strip (RBS).
- Table 13.1. Guidelines for establishing buffer strips in the western United States
- Retaining or Establishing Woody Debris
- Figure 13.4. General guidelines for the management of established riparian buffer strips
- Box 13.2 Definitions and Origins of Woody Debris
- Controlling Wildfires
- Rehabilitation Activities
- Meeting Instream Flow Requirements
- Other Considerations
- The Stream Continuum Model.
- The Concept of River Health.
- Influences of Riparian Communities on Flooding Events.
- Sustaining Buffer Strips
- Figure 13.5. Wetlands are characterized by the permanent or frequent presence of water and occur in a variety of inland and coastal land forms. Pictured is a black ash wetland in northern Minnesota
- Wetland Hydrology
- Hydrologic Conditions for Wetland Establishment
- Figure 13.6. Surface and groundwater relationships that form wetlands
- Water Budget of Wetlands
- Figure 13.7. Water budgets for a perched peatland bog and a groundwater-fed peatland fen. P, precipitation; ET, evapotranspiration; Q, surface discharge; ΔS, change in storage; GW, groundwater
- Evapotranspiration.
- Figure 13.8. Seasonal pattern of monthly streamflow from a perched bog and a groundwater-fed fen in northern Minnesota
- Figure 13.9. Streamflow duration relationships for a perched bog and a groundwater-fed fen watershed in northern Minnesota, each of about 53 ha area
- Groundwater Recharge.
- Figure 13.10. Relationship between saturated hydraulic conductivity (Ksat) and von Post H values of peat decomposition. The relationship for H4–H7 was determined by Gafni and Brooks (1990) and for H8–H10 from Päivänen (1973), as presented by Verry et al. (2011)
- Runoff and Streamflow from Wetlands.
- Figure 13.11. Cumulative frequency of occurrence of the water-table elevation at different peat horizons in a perched bog in northern Minnesota
- Figure 13.12. Streamflow from a peatland is governed by water-table elevation similar to the way that discharge from a reservoir is governed by pool elevation. Point 1 = high storage and elevation (peatland) correspond to high discharges (reservoir); point 2 = as water table (peatland) or pool (reservoir) drops, discharge decreases; point 3 = when respective elevations drop below the outlet (peatland) or spillway (reservoir), discharge ceases; z = datum
- Hydrologic Conditions for Wetland Establishment
- Concluding Thoughts – Hydrologic Functions of Wetlands
- Box 13.3 Streamflow Response from a Peatland Similar to that of an Unregulated Reservoir (from Verry et al., 1988)
- Figure 13.13. Relationship between peak-flow discharge (%) and percentage of a watershed in wetlands or lakes for different recurrence intervals in northern Wisconsin
- Cutting Trees
- Controlling Wildfires
- Prescribed Burning
- Mitigating the Effects of Roads
- Wetland Drainage for Economic Development
- Box 13.4 Proposals to Divert Water from the Sudd Wetland to Enhance Water Supplies in the Lower Nile River
- Wetland Drainage for Agriculture
- Box 13.5 Development Impacts on the Everglades of Florida, USA (from Enzler, 2012)
- Figure 13.14. The drainage of wetlands has removed excess water from a large portion of the prairie wetlands in the Minnesota River Basin for agricultural purposes
- Peat Mining
- Introduction
- Fragmentation of Watershed Landscapes
- Box 14.1 Fragmentation of Privately Owned Forests
- Conversion to Agricultural Croplands
- Table 14.1. Expected changes in hydrologic processes and responses resulting from the conversion of wildland watersheds (forests, grasslands) to agricultural croplands
- Streamflow Volumes and Regimes
- Water Quality
- Box 14.2 Hydrologic Changes due to Conversion of the Prairie Pothole Region to Annual Agricultural Cropping in the Upper Midwest (USA)
- Figure 14.1. Eutrophic water resulting from excessive nutrients from agricultural fertilizers applied in croplands
- Riparian Corridors and Wetlands
- Box 14.3 Water-Quality Impacts of Wetland Drainage and Vegetative Conversions in the Prairie Pothole Region of the Upper Midwest (USA)
- Figure 14.2. Two-stage ditch in southern Minnesota, USA, provides a buffer from cultivated land and roads
- Returning Agricultural Lands to Perennial Vegetation
- Box 14.4 Impacts of the Conservation Reserve Program (CRP) on Water Resources and the Environment
- Figure 14.3. CRP land croplands of southern Minnesota, USA. Perennial cover serves as wildlife habitat and functions to reduce nutrient and sediment loading to streams from adjacent croplands
- Conversion to Urban Developments
- Table 14.2. Changes in hydrologic processes and responses resulting from the conversion of wildland watersheds to urban developments
- Streamflow Regimes
- Water Quality
- Box 14.5 Effects of Urbanization on Stormflow: An Example
- Box 14.6 Monitoring Water Quality in a Municipality: One Example
- Riparian Corridors and Wetlands
- Box 14.7 Levees, Wetlands and Floodplains – An Example of Cumulative Effects
- Responses to Hydrologic Impacts Caused by Urbanization
- Water-Harvesting Systems
- Figure 14.4. An example of a simple water-harvesting system
- Catchment Areas
- Water Storage Facility
- Feasibility of Water Harvesting
- Synthesis of BMPs
- Effectiveness of BMPs
- Box 14.8 Best Management Practices: Forest Management for Protecting Water Quality and Wetlands (MDNR, 1995)
- Figure 14.5. Undesirable effects on soils and water such as illustrated in this photo can be avoided when BMPs are properly implemented. In this case, a perennial vegetated buffer would provide some degree of protection for the water quality in this stream
- Further Information on BMPs
- Federal Regulations
- National Environmental Policy Act
- The NEPA Process.
- Federal Agency Role.
- The Public's Role.
- Clean Water Act
- Box 14.9 A General Procedure for the Preparation of a TMDL
- Other Federal Regulations
- Safe Drinking Water Act.
- Endangered Species Act.
- Clean Air Act.
- Federal Insecticide, Fungicide, and Rodenticide Act.
- National Environmental Policy Act
- Impacts on Water Resources
- Figure 14.6. Snowpacks on watersheds in colder climates could be reduced with the initiation of snowmelt runoff earlier in the season with increased global warming
- Table 14.3. Observed water-related changes in regions of the United States during the last century
- Planning Protocols for Coping with Climatic Variability
- Use of Hydrologic Simulation Models
- Use of Stochastic Models
- Further Information on Climatic Variability
- Sources of Short-Term Information
- Obtaining Long-Term Information
- Box 14.10 Dilemma of Making Informed Decision with Insufficient Information: An Example of the Application of Dendrochronology
- Introduction
- Policies and Policy Processes
- A Policy and Its Components
- The Policy Processes
- Figure 15.1. The process of developing a policy for watershed management
- Identifying and Assessing Issues
- Defining Solutions and Proposing Policies
- Formalizing and Implementing Policies
- Box 15.1 Watershed-Related Problems and Policy Actions
- Monitoring and Evaluating Policy Implementation
- A Watershed Management Framework and Its Policy Implications
- Planning and Implementation
- An Overview of Planning for IWM
- Figure 15.2. The relationship of upland productivity (a) and sedimentation in a downstream reservoir (b) under conditions “with” and “without” watershed management practices
- Steps in the Planning Process
- Evaluating Past Activities and Identifying Problems and Opportunities
- Establishing Objectives, Identifying Constraints, Developing Strategies
- Identifying Alternative Ways to Implement the Strategy
- Box 15.2 A Single Objective versus Multiple Objectives
- Appraising Alternatives
- Making Appraisals Useful
- Table 15.1. Scope of effects of watershed management practices
- Risk and Uncertainty of Appraisals
- Making Appraisals Useful
- Recommending Action
- Planning as a Continuous Process
- An Overview of Planning for IWM
- Economic Appraisals
- Distinction between Economic and Financial Efficiencies
- Time Value of Money, Discount Rate, Present Value
- Steps in the Economic Appraisal Process
- Figure 15.3. Framework for examining relationships among physical effects, environmental changes and downstream benefits “with” watershed management practices as compared to conditions “without” the practices
- Identifying and Quantifying Physical Inputs and Outputs
- Table 15.2. Examples of inputs for a watershed management practice or project
- Valuing Inputs and Outputs
- Measures of Economic Value and Shadow Pricing.
- Developing Shadow Prices.
- Comparing Costs and Benefits: Measures of Project Worth
- Net Present Worth.
- Economic Rate of Return.
- A Benefit–Cost Ratio.
- Deciding Which Value Measure to Use
- Nonmonetary Benefits and Costs
- Application of the Economic Appraisal Process
- Proposed Watershed Management Activities
- Inputs and Costs
- Outputs and Benefits
- Valuing Benefits
- Comparing Costs and Benefits
- Results
- Introduction
- Generalized Hydrologic Simulation Models
- Development
- Spatial Representation
- Temporal Resolution
- Conceptual Basis
- Examples of Generalized Hydrologic Simulation Models
- Figure 16.1. Hydrologic processes and runoff relationships commonly found in generalized continuous simulation models
- Applications of Generalized Hydrologic Simulation Models
- Applications of Generalized Hydrologic Simulation Models on Forested Watersheds
- Forest Hydrology Simulation Models
- Desirable Characteristics
- Examples
- Development
- Remote-Sensing Platforms
- Optimal Remote Sensing
- Radar
- Geographic Information Systems
- Applications
- Figure 16.2. A GIS conceptualized as a set of geographically registered data layers
- Possible Errors
- Applications
- Global Positioning System
- Applications
- Errors
- Decision-Support Systems
- Linear Programming
- Multiple-Criteria Decision-Making Techniques
- Internet Applications
- Remote-Sensing Technologies
- Airborne and Satellite Remote-Sensing Techniques
- Light Detection and Ranging
- Advanced Geographic Information Systems and Geovisualization
- Multisensor Networks
- Stable Isotopes and Minnesota Lakes
- Table 16.1. Per mill change of δ18O values collected from Ten Mile Lake, Minnesota, between May 21, 2008, and October 11, 2010. Data show high amplitude of 0.71 and a low amplitude of 0.01, with an average amplitude change of 0.3%
- Figure 16.3. Isotope relationship of δD versus δ18O for lakes across Minnesota; although the data overlap, a pattern of data is present based on northern and southern lakes; with southern lakes (Prairie and Cornbelt, Transitional Forests) plotting toward the upper right and northern lakes (Canadian Shield) plotting toward the lower left of the graph. The line is the L, global meteoric water line (GMWL).
- Box 16.1 The Influence of Elevation on Stable Isotopes
- Box 16.2 Groundwater and Surface Water Exchange
- APPENDIX Units Commonly Used in Hydrologic Work, USA
- INDEX
- COLOR PLATES
- Plate 1. Measuring groundwater levels in a forested wetland with a pressure transducer in a shallow well
- Plate 2. Forested headwater watersheds are the source of most of the streamflow in the United States as depicted in this scene in the Northern Cascades of Washington
- Plate 3. Students measuring streamflow with a current meter
- Plate 4. The hydrologic cycle
- Plate 5. Unstable stream channels result in bluff erosion as depicted in the stream in southern Minnesota, USA
- Plate 6. Soil mass erosion can provide sediment to streams as depicted in this scene in southwestern Montana, USA
- Plate 7. A sediment splitter diverts progressively smaller fractions of streamflow to a collection tank to sample suspended sediment flowing through a rectangular weir in north-central Arizona. By also collecting bedload materials that settle in the catchment basin upstream of the weir total sediment yields can be measured from a watershed
- Plate 8. Comparison of the Channel Evolution Model (from Simon and Hupp, 1986) and the corresponding four Rosgen stream types
- Plate 9. The Rodeo-Chediski fire in Arizona, USA, burned more than 189 000 ha of mostly ponderosa pine forest in 2002, resulting in serious soil and water impacts
- Plate 10. Urbanization creates more surface runoff and compounds localized flooding – a 2011 scene of the Mississippi River at flood stage in St. Paul, Minnesota, USA
- Plate 11. Streamflow discharge peak-flow stage shortly after cessation of the Rodeo-Chediski Wildfire in a ponderosa pine watershed in Arizona; prefire peak-discharge stage noted
- Plate 12. A healthy riparian community is able to maintain an equilibrium between the streamflow forces acting to produce change and the resistance of vegetative, geomorphic, and structural features to the change. Riparian area in Honduras
- Plate 13. Wetlands are characterized by the permanent or frequent presence of water and occur in a variety of inland and coastal land forms. Pictured is a black ash wetland in northern Minnesota
- Plate 14. Undesirable effects on soils and water such as illustrated in this photo can be avoided when BMPs are properly implemented. In this case, a perennial vegetated buffer would provide some degree of protection for the water quality in this stream
UM RAFBÆKUR Á HEIMKAUP.IS
Bókahillan þín er þitt svæði og þar eru bækurnar þínar geymdar. Þú kemst í bókahilluna þína hvar og hvenær sem er í tölvu eða snjalltæki. Einfalt og þægilegt!
Rafbók til eignar
Rafbók til eignar þarf að hlaða niður á þau tæki sem þú vilt nota innan eins árs frá því bókin er keypt.
Þú kemst í bækurnar hvar sem er
Þú getur nálgast allar raf(skóla)bækurnar þínar á einu augabragði, hvar og hvenær sem er í bókahillunni þinni. Engin taska, enginn kyndill og ekkert vesen (hvað þá yfirvigt).
Auðvelt að fletta og leita
Þú getur flakkað milli síðna og kafla eins og þér hentar best og farið beint í ákveðna kafla úr efnisyfirlitinu. Í leitinni finnur þú orð, kafla eða síður í einum smelli.
Glósur og yfirstrikanir
Þú getur auðkennt textabrot með mismunandi litum og skrifað glósur að vild í rafbókina. Þú getur jafnvel séð glósur og yfirstrikanir hjá bekkjarsystkinum og kennara ef þeir leyfa það. Allt á einum stað.
Hvað viltu sjá? / Þú ræður hvernig síðan lítur út
Þú lagar síðuna að þínum þörfum. Stækkaðu eða minnkaðu myndir og texta með multi-level zoom til að sjá síðuna eins og þér hentar best í þínu námi.
Fleiri góðir kostir
- Þú getur prentað síður úr bókinni (innan þeirra marka sem útgefandinn setur)
- Möguleiki á tengingu við annað stafrænt og gagnvirkt efni, svo sem myndbönd eða spurningar úr efninu
- Auðvelt að afrita og líma efni/texta fyrir t.d. heimaverkefni eða ritgerðir
- Styður tækni sem hjálpar nemendum með sjón- eða heyrnarskerðingu
- Gerð : 208
- Höfundur : 6268
- Útgáfuár : 2012
- Leyfi : 379